Oxygen remains a critical consumable resource in medical disaster management and the primary therapeutic need for coronavirus disease 2019 (COVID-19) illness. Reference Ritz and Previtera1,Reference Blakeman and Branson2 At nearly every disaster, the logistics and planning for oxygen presents a substantial challenge. Recent hurricanes and cyclones (Hurricane Maria 2017, Typhoon Mangkhut 2018, and Cyclone Tauktae 2021), ice storms (Texas 2020), and firestorms (California 2020) have demonstrated a vulnerability of the power grid, which is a necessity for oxygen generation. When hospitals face a surge of overwhelming numbers of patients, alternative locations using repurposed buildings or tents in austere environments present inherent logistical and safety issues for medical oxygen. Previous alternate care site (ACS) plans for pandemics and disasters assumed that lower acuity patients would surge into gymnasiums, churches, stadiums, or schools for minimal supportive care until discharge. Reference Ritz and Previtera1,Reference Kobayashi, Hanagama and Yamanda3,Reference Devereaux4 However, the viral pneumonitis and acute respiratory distress syndrome (ARDS) caused by severe acute respiratory syndrome coronavirus 2 (SARS-CoV-2) in the current COVID-19 pandemic has resulted in patients who require high amounts of oxygen and equipment to help sustain them for a prolonged period of time. Oxygen support became one of the rate-limiting steps for medical care, hospitalizations, and patient discharges. In California, ACSs helped alleviate the strain from the surge of patients to hospitals, largely by providing oxygen to 1900 patients. Additionally, when hospitals depleted their oxygen and vendors could not re-supply them, the State needed to rapidly develop a large-scale oxygen support and delivery system. It is imperative for all responders to understand oxygen delivery, capacity, and logistical limitations. Reference Cupp and Predmore5 The unique and innovative multi-disciplinary clinical and logistical solutions by the state of California to meet the respiratory care needs of a population during a mass surge of COVID-19 patients is described in this study.
Background
During prepandemic conditions, clinicians in high-income countries assume an endless supply of oxygen from wall or concentrator systems; few have been trained on oxygen delivery in low resource environments. Due to the unprecedented high-flow oxygen demand used in caring for COVID-19 patients, many hospitals in the world have experienced rapid depletion of oxygen, resulting in significant mortality. Reference Pearson, Parkinson and Perez6–8 Available sources of oxygen include bulk liquid oxygen (LOX) systems, compressed gas cylinders, portable LOX systems, and oxygen concentrators. Plans for rapid deployment of oxygen and ventilators had significant limitations due to vendor supply, limited national inventory of portable steel oxygen cylinders, and inability to meet competing demands from multiple hospital systems. Moreover, hospital systems experienced decreases in oxygen pressure with limitations of flow due to multiple users of high-flow devices, flow through narrowed and outdated piping systems, and secondary freezing of external evaporators, valves, and other system components. Oxygen vendors only partially filled (rationed) liquid oxygen tanks for hospitals at this time and oxygen vendors were unable to fill LOX systems at hospitals that were not their regular customers, due to incompatible platforms or connectors. Table 1 provides a list of the most significant issues encountered during surge.
Table 1. List of issues faced during the COVID-19 pandemic regarding oxygen and ventilators
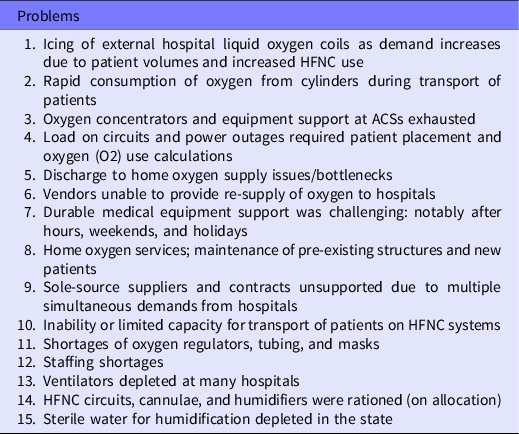
The infrastructure and knowledge needed to deliver oxygen and ventilators during a mass disaster or statewide emergency required novel solutions that had not been previously formulated or tested by California’s Emergency Medical Services Authority (EMSA). Equipment was rapidly procured, required testing, transport, and establishment of sites prepositioned near hospitals with the greatest needs. As part of the solution, California created an independent oxygen depot system of large capacity oxygen concentrators to supplement vendors and hospitals.
Discussion
Logistical Framework for Oxygen and Ventilator Equipment
The Disaster Management System
The California Office of Emergency Services (CAL-OES) is responsible for the overall coordination of the state’s response to disasters. EMSA is a department within the California Health and Human Services Agency that is responsible for coordinating the medical response to disasters in conjunction with the California Department of Public Health (National Emergency Support Function-ESF #8). EMSA provides direct medical services and logistics for medical response delivered by field teams that are comprised of volunteers mobilized as emergency temporary state employees through CAL-MAT (California Medical Assistance Teams). EMSA was tasked to provide support in the transfer, stocking, and emergent distribution of medical oxygen as well as other respiratory equipment. Before the pandemic, the EMSA equipment station contained a disaster inventory cache of fewer than 500 pieces of ventilatory equipment which grew to more than 30,000 items, comprised mainly of ventilators and supplies for oxygen support. This required a major expansion of warehouse capacity to approximately 280,000 square feet (26,000 square meters) for disaster response and management. The warehouse is also the logistics station with staff from EMSA, CAL-MAT, contracted medical staff, National Guard, and other first responders working together to meet supply requests.
Method to Request Oxygen
During a public health emergency, hospitals experiencing a deficiency of medical supplies submit requests to the county public health officer or regional medical and health operational area coordinator to see if the resource is available regionally. However, because the oxygen shortage was throughout the state and health systems did not have the means to share oxygen with 1 another, the requests were immediately directed to the state coordinating center (by means of a 24-h hotline created for oxygen requests) and to EMSA. While this seems circuitous, the process moved very rapidly. EMSA would then contact the facility and vendor that normally provides oxygen to the hospital and worked to supply the remaining amount. In some situations, the oxygen depot could provide all oxygen supply needs. The depot personnel prepared all aspects of the order, including paperwork, inventory, and delivery. Due to established contracts, hospitals were restricted from receiving supplies from noncontracted sources, thus requiring legal negotiation for state-supplied oxygen. Therefore, whenever possible, the state used established hospital–vendor relationships to provide emergency support. Follow-up contact ensured all critical needs were met.
Equipment and Maintenance- Biomedical Engineering and Warehousing
Deployment of equipment during medical surge to hospitals that are short-staffed and strained, requires the maximum external support deliverable. The state discovered that equipment emergently deployed from the Strategic National Stockpile (the United States’ depot of critical pharmaceuticals and medical supplies for emergencies) or even from manufacturers, often lacked maintenance, battery life, or component parts. Reference Branson, Dichter and Feldman9 Through a contract with a biomedical engineering team, EMSA restored deployable oxygen concentrators (DOCS) from long-term storage and deployed them to the oxygen depots, which is described further in the next section. Due to the age and condition of the DOCS systems, biomedical engineers were needed to inspect and test systems to ensure safety, full operation, and production of a minimum 93% pure oxygen (Figure 1). Each valve and connection underwent testing for leaks, all components were inspected for any damage or deterioration, and compressor coils were replaced as needed. This required work with the DOCS manufacturer to obtain necessary hoses and components for ultimate certification for deployment. The state was able to complete this process within 48 h following receipt of most equipment.

Figure 1. Ventilators being tested and run for 24 h at state warehouse.
The state also procured approximately 15,000 ventilators from 10 different manufacturers, with over half under federal emergency use authorization. Unfortunately, very few clinicians have expertise with this variety of new ventilators, that normally require hours of training and in-service by manufacturers when delivered to hospitals. Representatives from each manufacturer instructed a team that included biomedical engineers, a physician, a respiratory therapist (RT), and critical care nurses to understand the new ventilators that had been created under emergency use authorization. Not all of the ventilators delivered would meet the needs of a COVID-19 ARDS patient due to lack of full-feature capabilities. Reference Branson, Dichter and Feldman9 Newly acquired ventilators needed to be serviced and tested before they could be deployed because of the following:
-
1. The sudden demand for ventilators during a lockdown meant that most manufacturers did not have raw materials readily available to rapidly ramp-up manufacturing. Therefore, the ventilators that were provided often had parts that did not function or match the original specifications of the machine (ie, the lithium battery did not work resulting in a faulty ventilator or external flow sensors did not meet original specifications). All internal batteries were tested and the ventilator units run overnight to ensure proper operation and patient safety. Because the new ventilators under emergency use authorization still maintained a warranty, nonfunctional batteries on arrival were replaced by the manufacturer.
-
2. Approximately 400 of the newly procured ventilators were inoperable due to: alarm and sensor failure, low tidal volumes, or frozen touch screens. Because this scenario occurred repeatedly, the biomedical department collaborated with ventilator manufacturers, requesting precertification of each unit to ensure that ventilators were fully functional before arrival to the warehouse station.
Once the machines were properly serviced and tested, warehouse clinicians developed a 1-page quick start-up guide with diagrams for health-care workers who would be receiving a machine they had never operated. (These guides are available upon request).
Resource Tracking and Re-Supply System
Resource tracking and re-supply is a vital part of disaster preparedness. The state managed 5 separate ACSs supplying all logistics, medical equipment, most medications, oxygen concentrators, and additionally delivered over 2000 ventilators to numerous hospitals in need. In addition to the oxygen equipment and ventilators, the state also distributed humidifier systems, high-flow nasal cannula (HFNC) tubing, circuits, and connectors. Many of these assets were prestaged at the ACS for rapid delivery if needed by local hospitals during surge. Tracking of assets became a challenge with so many operations in progress simultaneously. Digitization of inventory with improved tracking and recovery was implemented to assure that state equipment such as field hospital tents, oxygen cylinders, and ventilators were not lost or stolen.
Establishment of Oxygen Depots
The State Oxygen Depot is a collaboration initiated by EMSA with CAL-OES, California Department of Public Health (CDPH), and local public health departments, to support hospital infrastructure and its need for oxygen. Reference Pierce, Osuna and Hodel10 California has 6 regions; each region would be provided with the same materials when requested. Four depots were strategically located to provide hospitals with oxygen if their liquid or compressed oxygen supply became low. Thirty-six individuals were deployed and received initial training at a central site, after which teams of 8 to 10 individuals would then staff each depot.
Each depot included at minimum: 2 stake bed trucks-used to transport H-cylinders, 1 DMSU (Disaster Medical Support Unit-loaded with D-cylinders), and 1 box truck for other supplies (Figure 2). Each stake bed truck carried a DOCS, Hospital Oxygen Back-up System (HOBS), 3 mass casualty bag manifolds, and 20 H-tanks with racks. Each DMSU contained oxygen regulators, oxygen concentrators, nasal cannulas, simple masks, nonrebreather masks, pulse oximeters, Venturi masks, and nebulizers with tubing. A diesel AC generator with a rating of 20 kW (25 kVA) was at every site with trained personnel operating all equipment to ensure adequate continuous power.

Figure 2. Oxygen depot assets.
The state has 9 DOCS systems with access to 3 others if needed. DOCS are deployable oxygen concentration systems that are commonly used to support field hospitals or used to fill cylinders that are then transported by truck to supply hospitals with oxygen. These machines typically have numbers associated with them (ie, DOCS 120, DOCS 200, DOCS 500), which represents the oxygen production flow rate in liters per min (L/min). A DOCS 200 discharges 200 L/min oxygen. A minimum of 1600 square feet (approximately 150 square meters) is required for the equipment and manifold for 2 DOCS-200 machines to operate and simultaneously fill oxygen tanks. The HOBS units are manifold systems that are used to fill oxygen H-tanks. It is an entirely passive system with no moving parts or power requirements. There is a single discharge port for delivery of low-pressure oxygen to a hospital supply line (Figure 2). The high pressure “H” cylinders, when fully pressurized, can supply approximately 8 h of oxygen at 120 L/min. This equates to continuously supplying 5 L/min to 24 patients simultaneously. Maximum flow rate is 240 L/min. Storage for up to 100 H-tanks in a cascade requires 15 feet (4.5 meters) by 20 feet (6 meters) of space.
The workspace requires adequate ventilation and should be adjacent to an outdoor location for the power generator to operate, receive fueling, and service. This location should have low public visibility, minimal traffic, and adequate access for ingress/egress of trucks or vehicles to drop and receive oxygen tanks. Ideally, this site should be located with proximity to highways or freeways for distribution. It is important to plan parking for vehicles, trucks, and forklifts to unload and load equipment from delivery trucks. Adequate ventilation, distance requirements for running generators or vehicles, and space with low traffic volumes will help eliminate the possibility of carbon monoxide contaminating medical oxygen. Security is needed for 24-h operations.
Oxygen Considerations at ACS
Peak COVID-19 patient volumes in California occurred from January to early February 2021 reaching over 1000 deaths daily and at 1 point, fewer than 50 hospital beds were available in a region serving 7 million people. Reference Patil11 This resulted in higher acuity patients requiring care at the ACS. Oxygen use became a criteria for admission to an ACS during hospital overflow in California (Supplementary Appendix 1). Care provided at each ACS was at a level equivalent to a general medical ward level, but facilities were gymnasiums, colleges, stadiums, or former residential care settings, with no medical gas piping. Patient census ranged from 20 to 50 patients at each of 5 sites located throughout the state (Christensen et al., publication pending). Hypoxemic patients requiring up to 10 L/min received oxygen by means of the Platinum-10 Invacare oxygen concentrators and home-fill systems. Over 500 units were procured by the state and deployed to the ACS locations. Some sites provided high-flow oxygen to a few patients, described in further detail below.
Goals of care included the provision of COVID-19 therapeutics, oxygen support, physical therapy, and weaning. RTs experienced in COVID-19 care were responsible for oxygen management and weaning at each ACS site, with aid from a team comprised of physicians, advanced practitioners, nurses, paramedics, emergency medical technicians (EMTs), and physical therapists, because the pandemic created staffing shortages of RTs. Oxygen scarcity at the ACSs became an issue from May 2020 to July 2020 and again from December 2020 to February 2021 and required oxygen conservation strategies described in further detail later in the article. The state also provided a home oxygen discharge program, managed by community paramedics and a home health nurse (Hutchinson H., publication pending).
Oxygen Systems
Oxygen Concentrators
Oxygen concentrators generate oxygen from ambient air by means of internal filters that absorb nitrogen, resulting in 90% oxygen concentration. Reference Hardavella, Karampinis and Frille12 Some stationary concentrators can generate up to 10 L/min of oxygen flow and work continuously for up to 1500 h of use. They depend upon a continuous power supply (up to 600W/concentrator*) and require regular maintenance and filter changes. Oxygen concentrators provide an advantage over use of tanks, as cylinders are rapidly depleted and require frequent monitoring and re-supply by staff (Supplementary Appendix 2), The main issue encountered while using oxygen concentrators in the ACS was the electrical demand; therefore, additional back-up power generators were in place due to frequent power failures when circuits were overloaded by patient usage. Power systems in some ACSs were old and unable to withstand the extra demand of use in hot-environment locations, such as the desert in Imperial County (peak summer high of 119° F/46° C). Reference Breyre, Sloane and Herring13 In the event of power failure, E-cylinders were placed at the foot of each bed and daisy-chained oxygen ports from H-tanks with mass casualty manifolds were strategically located to quickly connect patients. Circuit breakers required active monitoring due to overheating, and clinicians were advised to position patients according to electricity load required by oxygen flow rates. (Predicted loads for the Platinum 10 were based upon 585 W at 10 L/min flow rate, but electricity usage would be higher or lower depending upon flow and duration of use. Most concentrators have voltage and amperes printed on them. Watts can be calculated by multiplying V × A = W.
An additional emergency back-up generator was added to each ACS after power failures became a regularly occurring event and risk issue. All the deployed generators at each ACS were serviced every 10 d. Other vulnerabilities for power supply with the use of concentrators were energy use restrictions to preserve the power grid, rolling black-outs due to wildfire risks, and generator failures.
“Dual” Concentrators
Patient acuity was greater than initially planned at the ACS as hospitals retained the most critically ill patients and pressed the ACS to accept patients with higher oxygen needs. To provide adequate oxygen for acutely ill COVID-19 patients outside of hospital settings, dual oxygen concentrators were sometimes required. There is little literature to support dual concentrator application; however, direct communication with experts helped provide guidance. One concentrator was used for oxygen delivery with nasal cannula and the other used with either a simple face mask or a Venturi mask, depending upon the specific needs of the patient. For example, a simple mask with 10 L/min from 1 concentrator along with a nasal cannula rate of 6 L/min from another oxygen concentrator for a maximum of 16 L/min was commonly applied. For most patients, oxygen concentrators were in use for levels of 1-10 L/min using nasal cannulas, simple masks, or Venturi masks. Oxygen saturation monitoring and titration were used to achieve desired flow. As patients stabilized, they were weaned to a single concentrator with the oxygen device that most met their needs to maintain oxygen saturation (SpO2) >90%.
LOX Systems
LOX systems are the most common source of hospital oxygen. A liter of liquid oxygen evaporates to 860 L of gaseous oxygen, so a significant amount of oxygen can be stored and used from a smaller container. Portable LOX systems are available for hospital or tent use. A vessel evaporates the liquid through a series of vaporizing coils, similar to a radiator. In times of high demand, liquid oxygen at extremely low temperature (-183° C) can enter the vaporizing system and freeze the coils, regulators, or valves, causing the entire system to fail. The use of sprinklers with ambient temperature water and/or fans directed at the evaporators can reduce icing. Another technique is to rotate the vaporizing coils, but this requires 2 sets of coils. A LOX system can be placed where it is needed, and the lines can be plumbed to patient beds in an ACS or tent setting. This may be useful in resource or electricity challenged settings. One high acuity ACS in Imperial County established a simple system plumbing each patient bed in series, with tanks located outside for safety (Figure 3). This system can be easily rolled and stored when not in use and deployed for future emergencies. The local fire marshal inspected and approved the final set-up before patient-use. Planning for conversion from gas to liquid oxygen requires calculation so that the correct equipment is built and procured for use. This has been previously discussed in the literature. Reference Blakeman and Branson2,14 https://asprtracie.s3.amazonaws.com/documents/aspr-tracie-ta-oxygen-therapy.pdf.
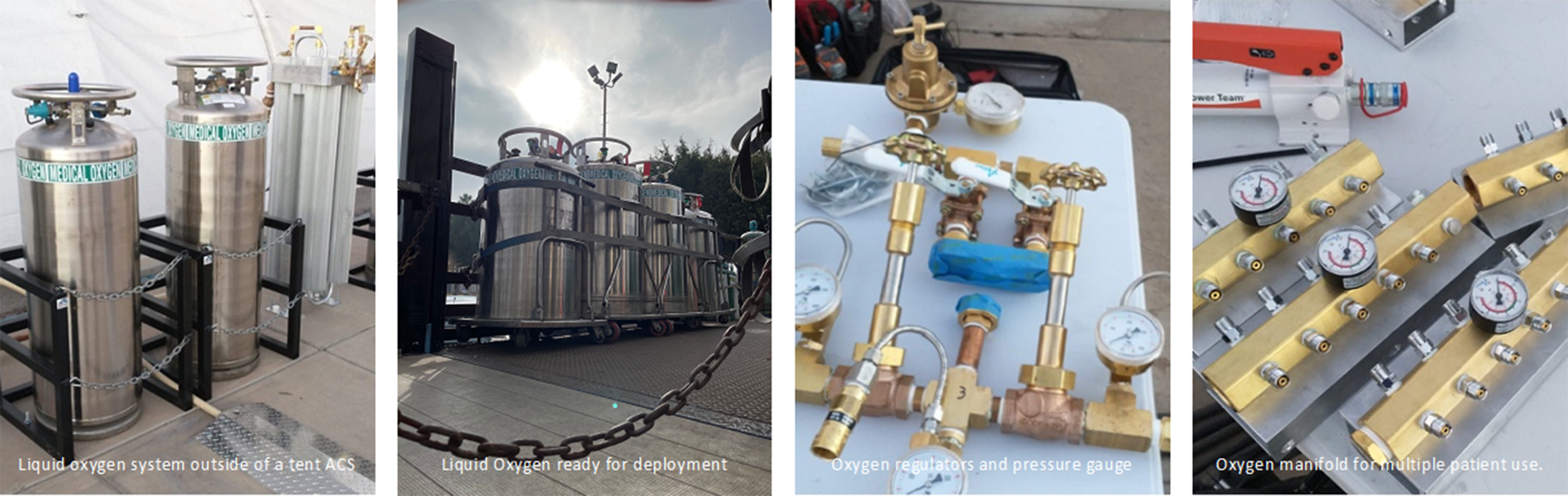
Figure 3. LOX system use at an ACS.
High Flow Nasal Cannula Experience in an ACS
HFNC oxygen is an oxygen system capable of delivering up to 100% humidified and heated oxygen at flow rates up to 60 L/min. Reference Nishimura15 Prepandemic, HFNC equipment was primarily used in intensive care units; however, during the pandemic, clinicians at every level, from EMTs to primary care, developed some familiarity due to patient surge and transportation needs. The use of HFNC caused rapid depletion of oxygen tanks and was used on a very limited basis in the ACS. No more than 1 person received HFNC at a time due to lack of oxygen supply and staffing. Humidification for HFNC also became a scarce resource, requiring a change from sterile water to distilled water. ACS patients on high-flow oxygen required an EMT at the bedside full-time, a diligent RT, and a nurse to provide oversight.
Weaning From Oxygen
Many countries lack RTs. With oversight and well-defined protocols in place, EMTs, paramedics, nurses, and advanced practice providers can successfully wean patients from oxygen. Reference Thakur16 Those on 4 L/min or less were managed by RT staff supervision of EMTs, paramedics, and nurses, only using provider consultation as needed. Those receiving more than 5 L/min of oxygen were managed by the RT with provider consultation (physician or physician assistant). All staff (EMTs, nursing aides, paramedics, nurses, RT, and physicians) raised oxygen flow rates if saturation was not above 90%. If the patient required greater than a 2 L/min increment in oxygen flow, staff alerted the RT and physician for an evaluation. All patients received humidification with their oxygen systems, unless unavailable.
Weaning included daily “oxy-walks”—ambulation with an oxygen cylinder and continuous oximetry monitoring to determine the safety of further weaning. Many patients required lower oxygen flows during sleep, but more while performing activities of daily living, such as toileting. Weaning was not performed in the evening to permit 1-2 h of observation before the night shift. This enabled stabilization if the patient failed daytime weaning before the night shift.
Home Oxygen Discharge Program
Difficulty in arranging oxygen and home monitoring for patients was frequently identified as a reason for limited hospital bed capacity, notably for those people who were under-insured or could not afford oxygen. To increase hospital bed availability, a home oxygen discharge program was established to expedite hospital and ACS patient discharges. Qualifying patients were greater than or equal to 18 y of age, required less than or equal to 4 L/min oxygen while at rest, clinically improving, with no other active medical problems requiring hospital treatment, and discharged from either the local ACS or hospital. Patients were provided with an oxygen concentrator, 2 oxygen E-cylinders, oxygen tubing, a nasal cannula, and an oxygen securement device. Daily in-home assessments were completed by local paramedics with the goal to decrease oxygen by 1 L/min flow, if clinically possible. The home oxygen program expanded care options and helped alleviate discharge bottlenecks in hospitals to provide care for more critically ill COVID-19 patients.
Oxygen Conservation (When Supplies Ran Low)
During times of oxygen conservation due to supply limitations, concentrators with long tubing were used instead of E-cylinders, which were typically used for patient mobility and toileting. Activities such as ambulating to the bathroom, going to the lounge or physical therapy were curtailed to conserve oxygen and was confined to in-room or local area rehabilitation. Tip sheets were created to help medical personnel conserve oxygen (Supplementary Appendix 3). At peak surge in California, when oxygen concentrators could not be procured, oxygen conserving devices (Oxymizers) were purchased to entrain and conserve oxygen delivered by means of nasal cannula. Consideration of splitting concentrators by use of Y-connectors for patients on lower flows became a back-up plan and was creatively used during India’s recent surge (Figure 4).

Figure 4. Creative use of Y connection for oxygen sharing.
In the United States, there is 1 authorized dual flow oxygen concentrator (Caire: The New Life Intensity), which is a 10-L unit that can be configured with two 5-L flowmeters. In several low- or middle-income countries, there are some other models of dual flowmeter units available. Because flowmeters are pressure compensated, if a kink occurs that is restrictive, flow would likely be diverted to the opposite side of the Y and create backpressure or flow reduction, which would be reflected on the flowmeter. To deliver oxygen to 2 patients with 1 concentrator, sufficient outlet pressures would be required; therefore, 10-L units which have 20 psi (pound per square inch) have been tested. Maximum tubing and cannula length of 57 feet (17 m) is advised. Due to low drive pressures used with most 5-L concentrators and lack of testing, it is not advised to share lower flow concentrators.
Emergency Medical Services Transports and Oxygen Conservation/Re-Supply
Transport by emergency medical services (EMS) during the COVID-19 surge posed new oxygen delivery challenges based on factors such as prolonged distance of transport, higher oxygen flow rates for severely ill patients, and increased use of air ambulance systems. To decompress overwhelmed hospital systems, hundreds of patients were transferred up to 600 miles using helicopter, fixed-wing aircraft, and ground ambulances to areas with staffed beds and supplies. Challenges during these transports included freezing of oxygen regulators and equipment during high-altitude transfers, sudden decompensation of ground-ambulance patients during travel over mountains due to altitude, and depletion of oxygen midway through transport due to unexpected higher flow rates needed to maintain oxygen saturation. When clinically appropriate, patients were transported while intubated, rather than on noninvasive ventilators, because oxygen flow rates were lower and freezing issues could be limited. For ground-transported patients traversing high-altitude routes, similar interventions used in the ACS were adapted for the field including having conscious patients self-prone in the ambulance, and using multiple complementary oxygen devices, eg, a nasal cannula and nonrebreather mask at high flow rates. When transports anticipated oxygen shortages, EMS supervisors would drive 1-2 h to meet units partway on the route with refills, often coordinating these restocking ventures with the receiving facility.
Oxygen Safety
Although oxygen itself is not a flammable gas, it is an oxidizer and enhances combustion. Materials that burn in air, such as clothing and bed linens, will burn fiercely in an oxygen-enriched atmosphere and require less energy to ignite. These materials can be ignited by a variety of sources such as smoking, open flames, friction, or expansion when oxygen at high pressure is rapidly introduced into a system that is initially at low pressure. A tip sheet was created so that all personnel working with oxygen would maintain safety protocols (Supplementary Appendix 4). The state also performed hospital site assessments to map emergency oxygen supply connections to ensure proper and safe equipment attachments in advance of need, notably due to these combustion risks. Reference Lydon17
Conclusion
Efforts continue by the State of California to mitigate hospital surge during COVID-19 and prepare for future disasters. Oxygen and ventilatory supply issues are a vital and important component to the program and requires learning about every step in the supply chain to deliver oxygen and ventilators safely to patients with respiratory needs. California has also worked with partners and other government agencies to share resources, including sending 500 ventilators by means of US Air Force cargo planes to New York and other impacted states in March 2020. In April 2021, a total of 240 oxygen tanks, 197 regulators, an DOCS 200 with booster, and 43 mass casualty oxygen manifolds were deployed within 24 h of request to assist India. 18 It is hoped that this information will assist others to rapidly deploy oxygen delivery systems during any mass patient care situation and to anticipate the necessary logistics required to effectively care for patients requiring respiratory support.
Acknowledgments
Thanks to Craig Johnson, Michael Frenn, Gerald Osuna, Jared Hodel, David Rios Jr., Bryan Levine, Camie Wiltshire, Clayton Boyer, Brad Gates, Brandon Benz, Randy Hania, and Marguerite Vardman. The acknowledgements recognize the tremendous work, organization, and leadership by individuals at EMSA and CAL-MAT who implemented the logistics of oxygen delivery. The authors also recognize the numerous volunteer responders who worked throughout California during the COVID-19 pandemic and state fire responses of 2020.
Author contributions
All authors contributed to the development of the manuscript and provided content for this article.
Conflicts of interest
Richard Branson reports relationships with Mallinckrodt, Pfizer, Ventec, Vyaire, and Zoll. His contributions to the study dealt with oxygen conservation and did not influence any decisions made by the state.
Supplementary material
To view supplementary material for this article, please visit https://doi.org/10.1017/dmp.2021.267