Commercial cigarettes consist of a specially prepared tobacco blend, air-permeable paper and a common filter made of cellulose acetate fibres. In addition, several substances are added to tobacco blends to impart specific desirable properties (Thielen, Reference Thielen, Klus and Müller2008). Cigarettes can be burned either with flame or flameless during smoking. As tobacco is a natural organic material, the smoke formed through flame burning contains mainly carbon dioxide, carbon monoxide, nitrogen oxides and water vapours. The cigarette smoke is essentially an aerosol composed of liquid droplets suspended in a mixture of these gases and water vapour. Moreover, during the smoking process, two kinds of smoke with various physicochemical properties are released. The first is mainstream smoke (MSS) inhaled by the smoker. The second is sidestream smoke (SSS) released into the environment between puffs from the flameless burning at the other end of the cigarette. The formation temperature intervals for the MSS and the SSS are 850–950°C and 500–650°C, respectively (Thielen, Reference Thielen, Klus and Müller2008).
The temperature of the MSS decreases gradually along the direction towards the smoking end of the cigarette. Numerous toxic compounds found in the MSS originate from burning, pyrolysis and pyrosynthesis between the two ends of the cigarette (Rustemeier et al., Reference Rustemeier, Stabbert, Haussmann, Roemer and Carmines2002; Counts et al., Reference Counts, Morton, Laffoon, Cox and Lipowicz2005; Panday & Kim, Reference Panday and Kim2010). The condensed residue in the filter materials is a mixture of tar, which contains various toxic polycyclic aromatic hydrocarbons, and alkaloids, mainly nicotine.
The International Union of Pure and Applied Chemistry (IUPAC) name for nicotine (C10H14N2) is 3-(1-methyl-2-pyrrolidinyl) pyridine and its structural formula is as follows:
The solubility of nicotine in water and organic solvents varies depending on the pH value of the mixture. Nicotine has a standard boiling temperature of 245.5°C and dissolves in water completely at room temperature. However, the mixture of water and nicotine releases emulsions at a temperature range of 60–210°C depending on the composition at atmospheric pressure. By contrast, the large organic compounds in tar are insoluble in water.
Some of the toxic compounds in the MSS are removed using a filter tip, which facilitates several physicochemical processes such as condensation, adsorption, absorption and various chemical reactions (Smith et al., Reference Smith, Livingston and Doolittle1997; Ködderitzsch et al., Reference Ködderitzsch, Bischoff, Veitenhansl, Lorenz and Bischoff2005; Purkis et al., Reference Purkis, Cahours, Rey, Teillet, Troude and Verron2011). However, most of the common cigarette filter tips alone do not fully remove the compounds that are harmful to human health (Kim et al., Reference Kim, Kabir and Jahan2016; Pinto et al., Reference Pinto, Cruz, Ramos, Santos and Almeida2017). To increase the efficiency of filters, several materials such as silk fibres (Tian et al., Reference Tian, Chen, Chen and Bai2009), alumina (Verdolotti et al., Reference Verdolotti, Salerno, Lamanna, Nunziata, Netti and Iannace2012), zeolite (Xu et al., Reference Xu, Zhu, Ma, Ji, Wei and Shang2003; Gao et al., Reference Gao, Cao, Zhou, Zhuang, Wang and Zhu2009), active carbon (Coggins et al., Reference Coggins and Gaworski2008; Sasaki et al., Reference Sasaki, Matsumoto and Yamashita2008), carbon nanotubes (Chen et al., Reference Chen, Zhang, Tang and Jia2006), carbon nanocages (Li et al., Reference Li, Yu, Xu, Ma, Chen, Hao and Qian2011) and sepiolite (Zhang Reference Zhang2000) have been added to the filter tips (Lamb & Parenski, Reference Lamb and Parenski1984; Figlar et al., Reference Figlar, Tucker and St. Charles2004). In previous studies, sepiolite granules with diameters of ≤100 μm were admixed with cigarette paper or were distributed in the cellulose acetate filter tip (Artho et al., Reference Artho, Beringer, Buchman and Koch1976). However, it is still unclear how important the granule size and necessary mass of the inorganic additives are for sufficient removal of tar and nicotine.
Among the porous materials used to increase the efficiency of smoke filtration, only sepiolite has a fibrous crystal structure (Moore & Reynolds, Reference Moore and Reynolds1997; Bergaya et al., Reference Bergaya, Theng and Lagaly2006). The natural sepiolite clays typically contain carbonates such as dolomite (CaMg(CO3)2), calcite (CaCO3), magnesite (MgCO3) and other impurities depending on the deposit and geological origin (Inukai et al., Reference Inukai, Miyawaki, Tomura, Shimosaka and Irkeç1994; Yener et al., Reference Yener, Önal, Üstünışık and Sarıkaya2007). It is also possible to synthesize pure sepiolite using MgCl2 and SiO2 mixtures under laboratory conditions (Abtahi, Reference Abtahi1985). The chemical formula of pure sepiolite is Si12O30Mg8(OH)4(OH2)4.8H2O for the half-unit cell (Brauner & Preisinger, Reference Brauner and Preisinger1959; Serna et al., Reference Serna, Ahlrichs and Serratosa1975; Tian et al., Reference Tian, Han, Wang, Liang, Wang and Wang2019), where (OH)4, (OH2)4 and 8H2O represent the structural hydroxyls (Ahlrichs et al., Reference Ahlrichs, Serna and Serratosa1975; Yılmaz et al., Reference Yılmaz, Kalpaklı and Pişkin2013), bound water (Kıyohiro & Otsuka, Reference Kıyohiro and Otsuka1989; Ogorodova et al., Reference Ogorodova, Kiseleva, Vigasina, Kabalov, Grishchenko and Mel'chakova2014) and zeolitic water (Prost, Reference Prost1975; Rouquerol et al., Reference Rouquerol, Rouquerol, Sing, Llewellyn and Maurin2014), respectively. The zeolitic water of sepiolite is removed by heating to 250°C (Bergaya et al. Reference Bergaya, Theng and Lagaly2006; Sarıkaya et al. Reference Sarıkaya, Önal and Pekdemir2020b). Accordingly, the nanopores in air-dried sepiolite are filled with zeolitic water.
The crystal structure and nanoporosity of sepiolite can be modified easily using thermal treatments depending on the temperature conditions applied (Balcı, Reference Balcı1999; Frost & Ding, Reference Frost and Ding2003; Perraki & Orfanoudaki, Reference Perraki and Orfanoudaki2008; Wang et al., Reference Wang, Liang, Tang, Chen and Chen2014). Sintering of sepiolite at higher temperatures produces new phases (Kulbicki, Reference Kulbicki1959; Göktaş et al., Reference Göktaş, Mısırlı and Baykara1997). The kinetics and thermodynamic parameters for the thermal degradation of sepiolite at various applied temperatures have been evaluated using X-ray diffraction (XRD) and thermal analysis (Sarıkaya et al., Reference Sarıkaya, Önal and Pekdemir2020a, Reference Sarıkaya, Önal and Pekdemir2020b).
Raw and modified sepiolite samples have found various applications (Galan, Reference Galan1996; Murray, Reference Murray1999): for example, as adsorbents (González-Santamaria et al., Reference González-Santamaria, Lopez, Ruiz, Fernández, Ortega and Cuevas2017), clarification agents (Erdoğan et al., Reference Erdoğan, Demirci and Akay1996; Mirzaaghaei et al., Reference Mirzaaghaei, Goli and Fathi2017), bleaching earths (Tian et al., Reference Tian, Wang, Kang and Wang2014; Laatikainen et al., Reference Laatikainen, Srithammavut, Toukoniitty, Turunen and Sainio2015; Saneei et al., Reference Saneei, Goli and Keramat2015) and catalysts (Al-Ani et al., Reference Al-Ani, Gertisser and Zholobenko2018; Meşe et al., Reference Meşe, Figen, Filiz and Pişkin2018). Acid activation (Çetişli & Gedikbey, Reference Çetişli and Gedikbey1990; Yebra-Rodriguez et al., Reference Yebra-Rodriguez, Martin-Ramos, Del Rey, Viseras and Lopez-Galindo2003) and other chemical modifications (Shuali et al., Reference Shuali, Yariv, Steinberg and Müller-Vanmoos1991; Fitaroni et al., Reference Fitaroni, Venâncio, Tanaka, Gimenez, Costa and Cruz2019; Papoulis et al., Reference Papoulis, Somalakidi, Todorova, Trapalis, Panagiotaras and Sygkridou2019) have a strong effect on the physicochemical properties of sepiolite, including on its crystal structure (Özdemir & Kıpçak, Reference Özdemir and Kıpçak2004), surface acidity (Bonilla et al., Reference Bonilla, Lopez-Gonzales, Ramirez-Saens, Rodriguez-Reinoso and Valenzuela-Calahorro1981), surface area (Jimenez-Lopez et al., Reference Jimenez-Lopez, Lopez-Gonzalez, Ramirez-Saenz, Rodriguez-Reinoso, Valenzuela-Calahorro and Zurita-Herrera1978), nanoporosity (Rodriguez-Reinoso et al., Reference Rodriguez-Reinoso, Ramirez-Saenz, Lopez-Gonzalez, Valenzuela-Calahorro and Zurita-Herrera1981) and catalytic activity (Corma et al., Reference Corma, Perez-Pariente, Fornes and Misfud1984). The purpose of this study was to investigate the effects of mean particle size and the added mass of sepiolite granules as a filter additive for improving the removal of tar and nicotine in the MSS of cigarettes based on controlled experimentation.
Material and methods
Sepiolite from Eskişehir, Turkey
The white-coloured sepiolite, also known as pipestone, was used as an additive in cigarette filter tips. Its geological formation and mineralogical composition have been extensively investigated in previous studies (Ece & Çoban, Reference Ece and Çoban1994). A number of its physicochemical properties (chemical composition, mineralogy, morphology, particle-size distribution, porosity and specific surface area) as well as the change in its crystal structure and linear shrinkage as a function of the temperature of the raw sepiolite used in this study have been examined previously (Önal et al., Reference Önal, Yılmaz and Sarıkaya2008). The chemical composition of the sepiolite in mass% is: SiO2, 55.45; MgO, 26.53; Al2O3, 0.85; Fe2O3, 0.07; TiO2, 0.06; CaO, 1.86; Na2O, 0.06; K2O, 0.08; and loss on ignition (LOI), 14.60. The sepiolite contains 6.3 mass% dolomite and has a cation-exchange capacity (CEC) of 0.27 meq g–1. The internal widths of the voids in solids <2 nm, between 2 and 50 nm and >50 nm are called micropores, mesopores and macropores, respectively (Rouquerol et al., Reference Rouquerol, Rouquerol, Sing, Llewellyn and Maurin2014). In addition, the voids of interval width <100 nm are defined as nanopores. Sepiolite has open intra-crystalline pores in the form of interconnected channels running parallel to the fibre length (Bergaya et al., Reference Bergaya, Theng and Lagaly2006). The specific micropore, mesopore and macropore volumes for the sepiolite used are 0.16, 0.21 and 0.45 cm3 g–1, respectively. The outer and inner specific surface areas of sepiolite are 8 and 250 m2 g–1, respectively. Therefore, the adsorption capacity of the outer surface of the sepiolite is negligible compared to that of the inner surface.
The sepiolite was ground gently in an agate mortar after drying at room temperature for 1 day. The obtained shapeless granular material was then separated into seven size fractions by dry sieving. The upper, lower and mean grain sizes of the fractions (–r, +r and r, respectively) are listed in Table 1. Each fraction was stored in a plastic bottle with the lid closed tightly. The crystal structure, chemical composition, open porosity and adsorption capacity were the same in all of the size fractions. Only the fluid permeability changed depending on the mass, number, size and shape of the granules. The void volume distribution between the shapeless sepiolite granules was almost same as their particle-size distribution.
Table 1. The dry screen analysis of sepiolite granules.
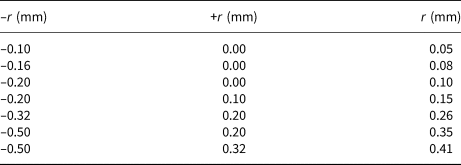
Cigarettes
Commercial cigarette samples 8 mm in diameter, 85 mm long and with 20 mm cellulose acetate filter tips were selected according to standards of the Cooperation Centre for Scientific Research Relative to Tobacco (CORESTA). Unfiltered cigarettes were prepared by carefully removing the filter tips. Various precursors were obtained by removing 5 mm-long pieces from the middle of cellulose acetate matrix (Fig. 1). Modified filters were prepared by packing the sepiolite granules of a mean particle size into the void volume by a given amount. The prepared precursors include unfiltered (UF), cellulose acetate-filtered (CAF), precursor-filtered (PF) and sepiolite modified-filtered (SMF) cigarettes (Fig. 1). All cigarettes were kept at 22°C and 65% relative humidity for 48 h prior to machine smoking.

Fig. 1. Schematic representation of the UF, CAF, PF and SMF cigarettes.
Machine smoking
Five cigarettes for each sample (UF, CAF, PF and SMF) were smoked by using a Filtrona Model 302. A pad (Filtrona) 44 mm in diameter and 2 mm thick was used as a machine filter. During the smoking test, the parameters of the machine were set to run each experiment for 60 s with 2 s puff intervals, a puff volume of a 35 mL and a duration of 10 s between consecutive puffs. The butt lengths of the unfiltered and filtered cigarettes were kept at 8 and 28 mm, respectively.
Smoke condensate
A heterogeneous mixture of water, nicotine and tar trapped on the machine filter is referred to as the crude-smoke condensate (CSC). The machine filter and its accessories were weighed before and after the smoking process. The difference between the two values obtained was taken as the mass of the CSC. The CSC was dissolved completely in 100 mL of methanol and the water was removed by selective adsorption on a dried synthetic zeolite 4A molecular sieve with high adsorption capacity. Nicotine and larger hydrocarbon molecules in the tar did not penetrate the 0.4 nm-wide zeolite nanopores and remained in the solution. The water content in the CSC was considered equal to the mass increase of the zeolite 4A due to adsorption. The difference between the masses of the CSC and water was then considered as the content of the dry-smoke condensate (DSC). The results were calculated as the mass of the CSC, water and DSC per cigarette filter.
Nicotine and tar
The anhydrous methanol solution contains nicotine and various types of organic molecules present in tar. This solution was mixed with a sufficient amount of water in a steam distillation flask. Alkaline nicotine was first converted into non-volatile sulfate salts by adding 5 mL of 1.00 M H2SO4 solution according to equation (1):

The remaining volatile organic compounds originating from tar were then removed by steam distillation.
The nicotine sulfate salts remaining in the flask after steam distillation were again converted into nicotine molecules by adding 25 mL of 2.00 M NaOH solution according to equation (2):

Nicotine was purified by steam distillation. The nicotine content was determined with an ultraviolet–visible light (UV-VIS) spectrophotometer (Varian, Cary 50) at the wavelengths of maximum absorption (236, 259 and 282 nm). The tar content was calculated as the difference between the masses of DSC and nicotine content. The masses of nicotine content and tar content retained in the machine filter from the MSS were calculated for each cigarette.
Examination of used filter tips
Scanning electron microscopy (SEM) and energy dispersive X-ray spectroscopy (EDX) analyses of the sepiolite samples before and after use as additives in the cigarette filter tips were obtained using a QUANTA 400F Field Emission SEM instrument operating at 30 kV. Prior to analysis, all samples were mounted on aluminium tape and coated with gold. The Fourier-transform infrared (FTIR) spectra of the raw and used sepiolite granules were obtained using a Spectrum 100 Perkin Elmer FTIR spectrometer. The FTIR spectra, in the range 400–4000 cm–1, were obtained using the attenuated total reflection sampling technique. For each sample, ten scans were recorded with a resolution of 10 cm–1. The XRD traces of the same samples were then recorded after preparation of oriented mounts following the glass slide method (Moore & Reynolds, Reference Moore and Reynolds1997) and using a Rigaku D-Max 2200 Powder Diffractometer with Ni-filtered Cu-Kα radiation at 40 kV and 30 mA and a scanning speed of 2°2θ min–1.
Results and discussion
Removal yields of the cigarette filters
The mass balance of the condensate formed from the MSS per filtered cigarette can be written as follows:

where m u is the total mass, m f is the mass removed by the cigarette filter and m m is the trapped mass on the machine filter (Fig. 1). Thus, the mass balance for an unfiltered cigarette is as follows:

The yield (y) of the condensate removed from the MSS using a filter tip is defined as follows:

The calculated masses and removal yield of tar and nicotine in the MSS with the CAF and PF cigarettes are given in Table 2.
Table 2. Removal yields of tar and nicotine in the MSS by the UF, CAF and PF cigarettes.
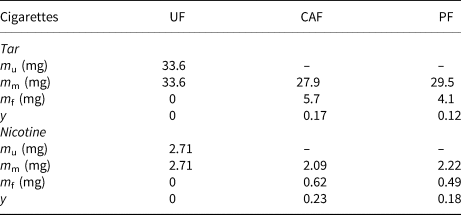
Relationship between the removal yield and particle size of sepiolite
The SMF cigarettes (Fig. 1) smoked by the machine differ in the granule-size distribution of sepiolite. Accordingly, the process variable is mean particle size (D). The variation in the removal yields (y) of tar and nicotine as a function of D for 95 mg of sepiolite granules is shown in Fig. 2. The yields that are constant in the range of 0.15 mm < D < 0.41 mm reached a maximum value at D = 0.10 mm. The difference in removal yields for the given size interval of D was due to the smaller nicotine content in the MSS compared to that of tar and partly to the dissolution of nicotine in the zeolitic water. The condensation increased at D < 0.10 mm due to the decrease in the permeability of larger organic molecules among the fibre clusters of sepiolite. Consequently, cellulose acetate cigarette filter can be modified to obtain a desirable removal yield for tar and nicotine by changing the mass of the sepiolite granules with D = 0.10 mm.
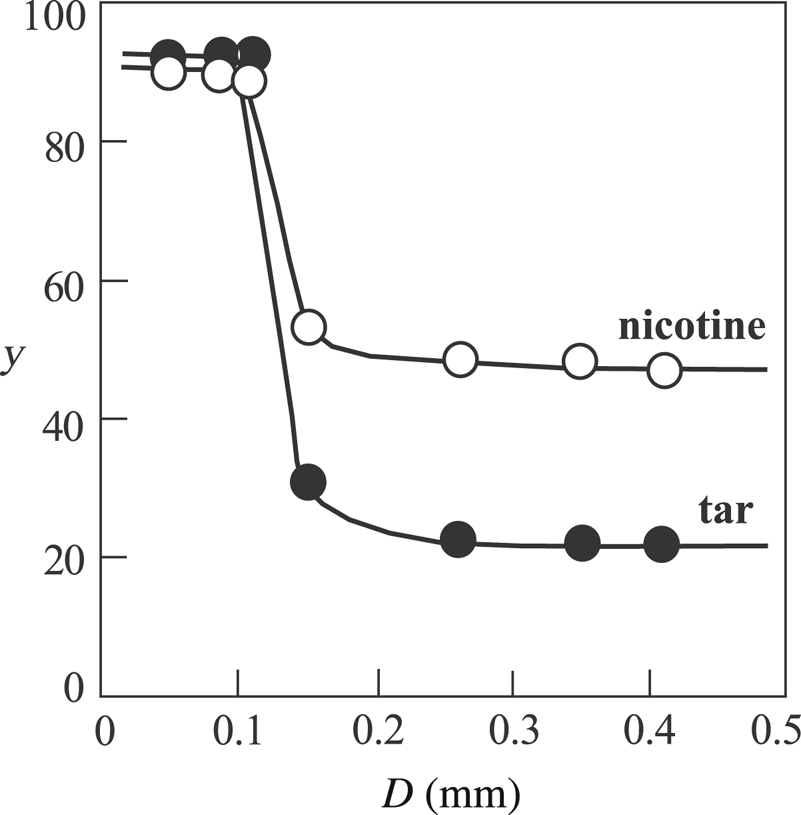
Fig. 2. The variation of the removal yields (y) for tar and nicotine with respect to the granule size of sepiolite with a mass (m s) of 95 mg.
Relationship between the removal yield and mass of sepiolite
The SMF cigarettes (Fig. 1) smoked by the machine are similar except for the mass of sepiolite. Accordingly, the variable of the process is the mass of the sepiolite granules with a similar particle-size distribution. The variation in the removal yields (y) of tar and nicotine with respect to the mass of the sepiolite granules with a D of 0.10 mm is given in Fig. 3. Despite the different masses of tar and nicotine retained per cigarette filter, the corresponding removal yields were almost equal in both cases. The yields increased gradually with the increasing mass of sepiolite granules of D = 0.10 mm, showing a smooth curve. Thus, filter tips with the desired removal yields for tar and nicotine can be prepared by modifying the cellulose acetate filter using sepiolite granules with definite mean particle sizes and masses.
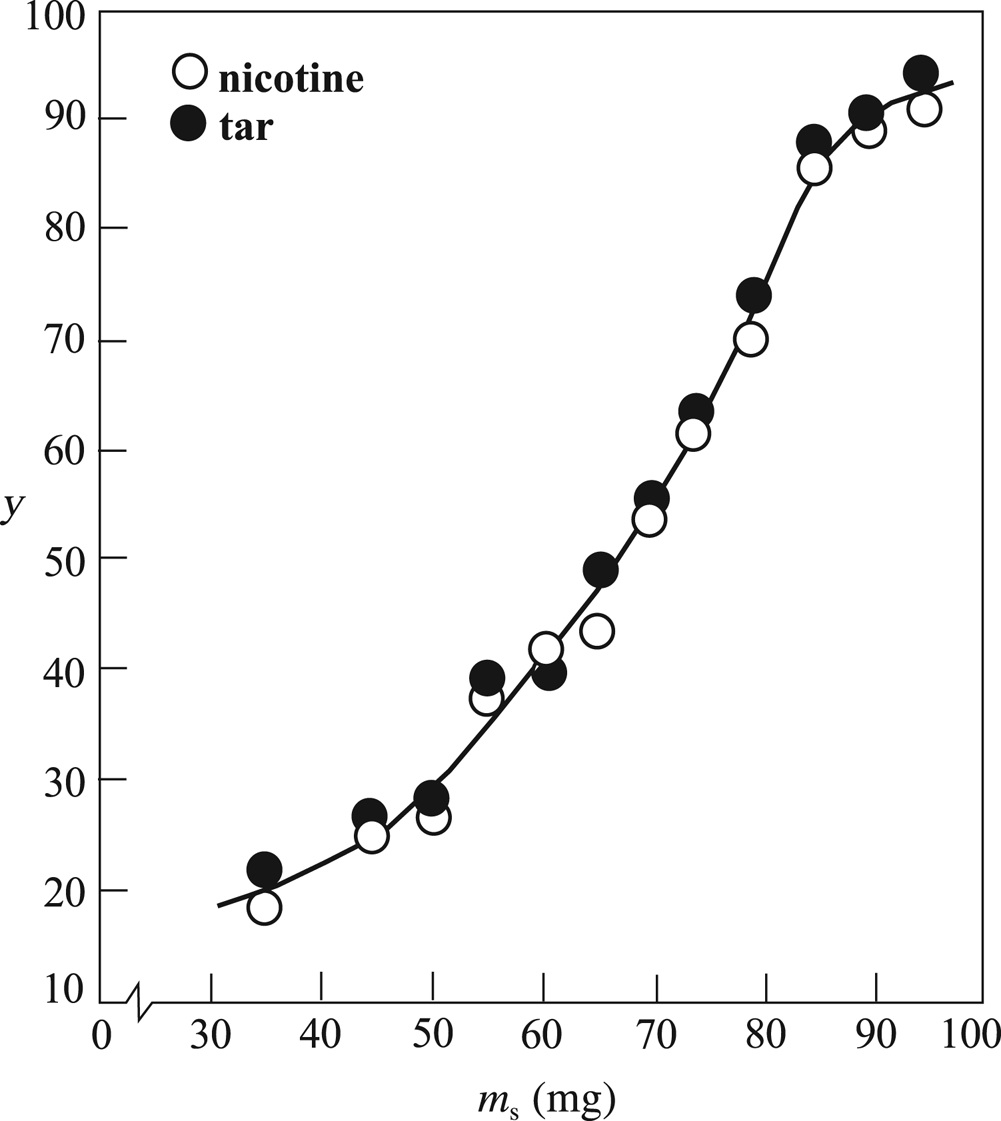
Fig. 3. The variation of the removal yields (y) of tar and nicotine with respect to the mass of the sepiolite (m s) of a mean granule size (D) of 0.10 mm.
Interaction between sepiolite and cigarette smoke
The removal yields of sepiolite granules at the same mass depend on the size distribution. In addition, they depend on the number of the granules having different masses but similar size distributions. The condensation amount increases with both the size and mass of the granules, whereas the adsorbed amount is affected only by the mass and not by the size of the granules. Hence, the removal of tar and nicotine by the sepiolite granules is due to condensation rather than adsorption. Condensation occurs during the MSS flow through the stationary bed of rough sepiolite granules of different sizes, shapes and numbers that are randomly compacted. The flow rate decreases with the increasing number of collisions amongst the large organic molecules and the granules. Hence, the friction during the MSS flow through the bed of sepiolite granules increased the retention time and trapped the condensate.
The SEM images show the fibre cluster structure of sepiolite granules and the condensation of large molecules in the tar and nicotine on the fibres of the clusters (Fig. 4). The EDX spectra of the raw and used sepiolite samples are shown in Fig. 5. The excess amount of carbon in the used samples shows that the accumulation on the sepiolite bed is due to condensation rather than adsorption. The remaining gaseous mixture flows through the voids between the granules. The FTIR bands on the spectrum of raw sepiolite (Perraki & Orfanudaki, Reference Perraki and Orfanoudaki2008) and used sepiolite (Fig. 6) are also in agreement with the SEM images. The stretching bands for C–H, C=C, C=N and CH3–N indicate that the tar and nicotine were mainly condensed on the sepiolite fibres after multimolecular adsorption. According to the XRD traces, the smoke does not affect the crystal structure of the sepiolite (Fig. 7).
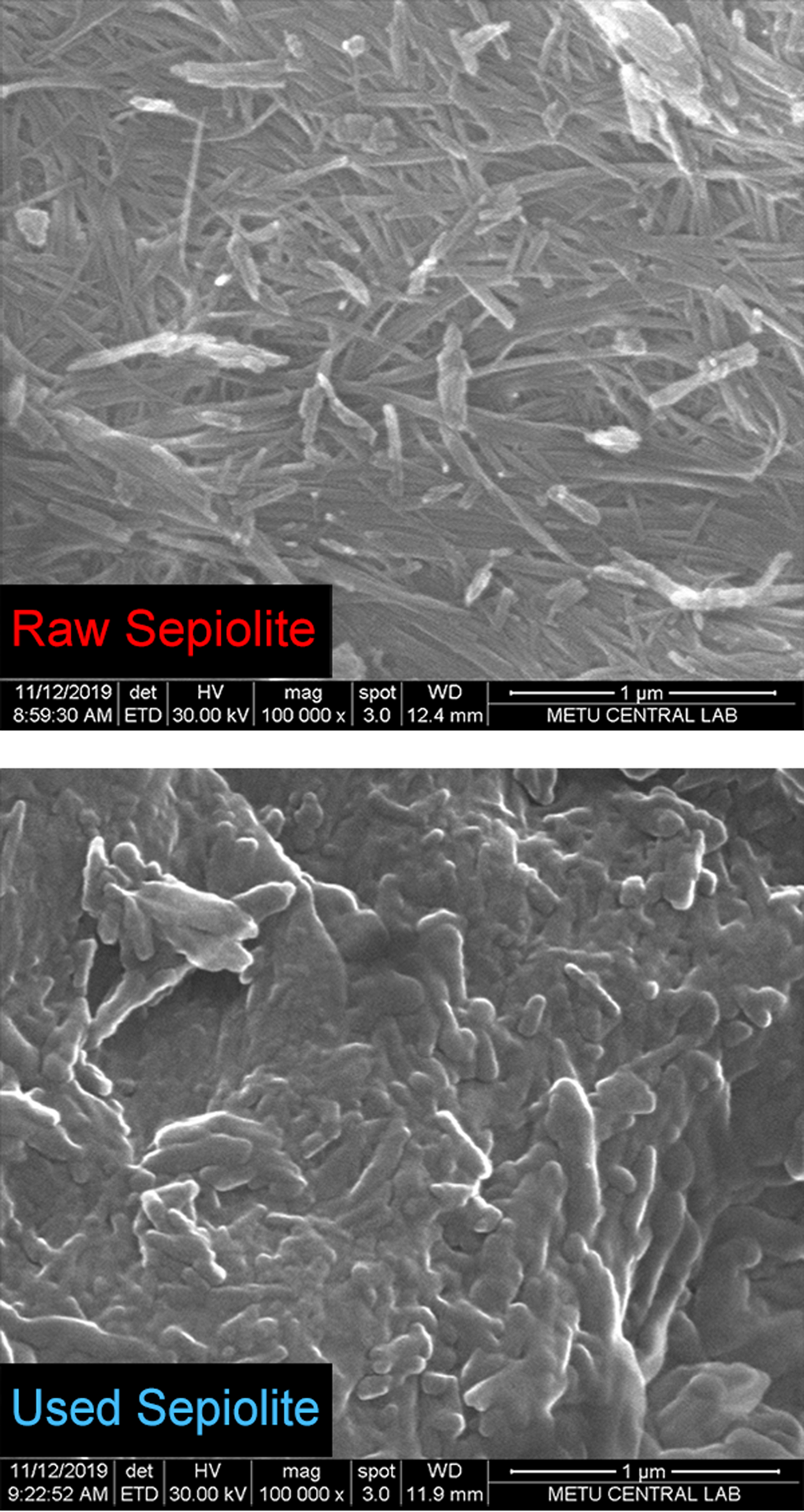
Fig. 4. SEM images of the sepiolite samples.
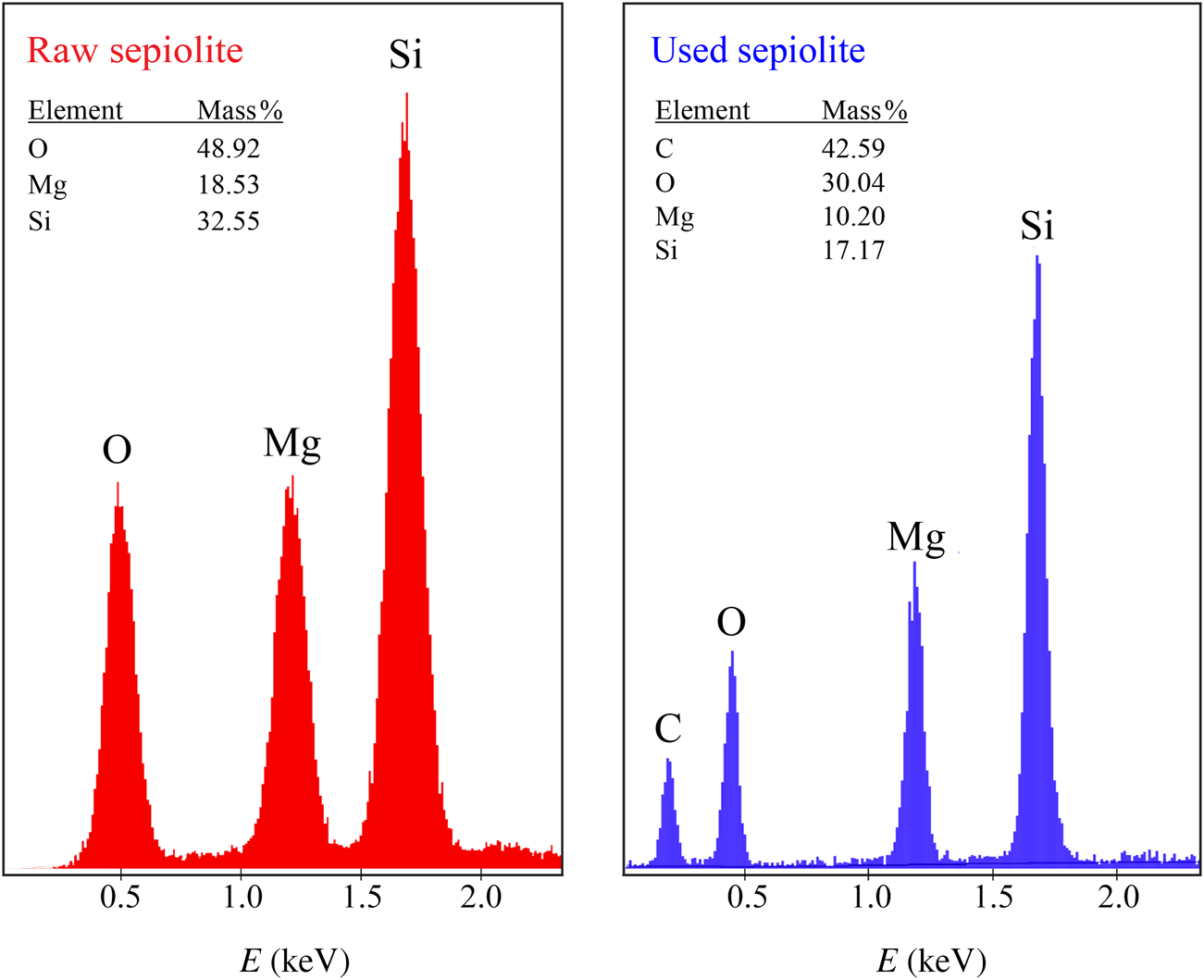
Fig. 5. EDX spectra of the sepiolite samples.
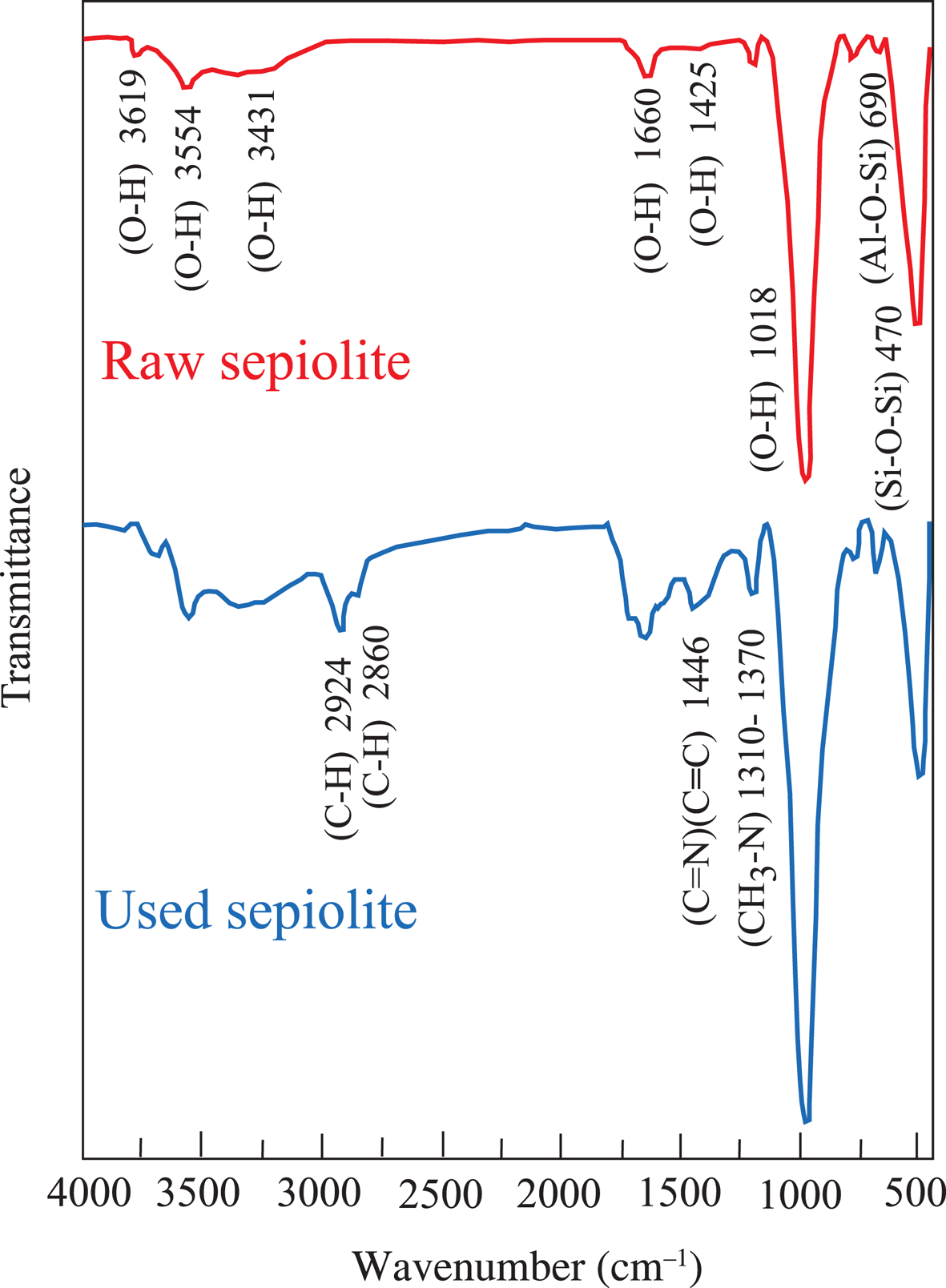
Fig. 6. FTIR spectra of the sepiolite samples.
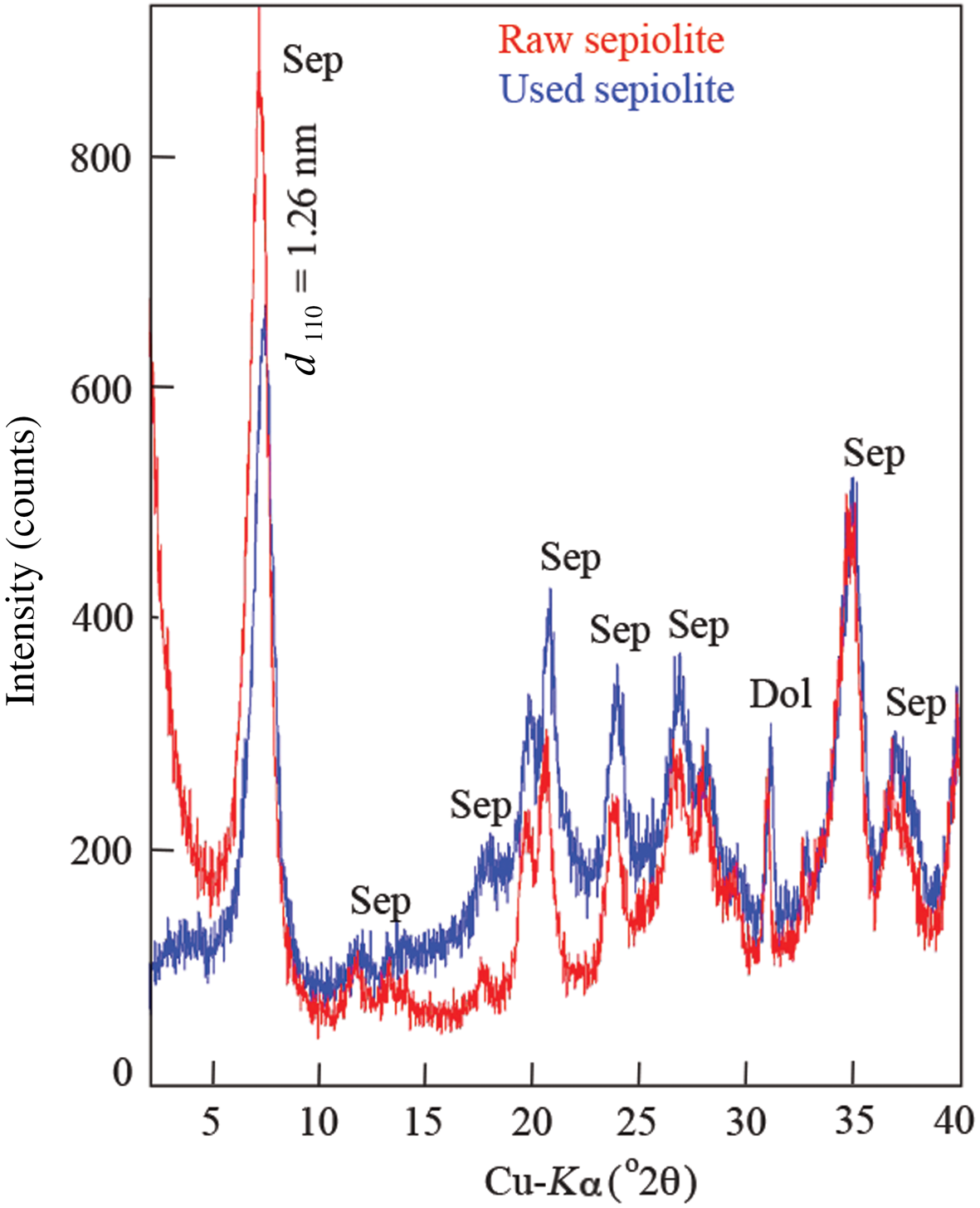
Fig. 7. XRD traces of the sepiolite samples. Sep = sepiolite; Dol = dolomite, according to Warr (Reference Warr2020).
Conclusions
The air-dried sepiolite granules with zeolitic water were effective additives in cellulose acetate filter tips for the removal of tar and nicotine from the MSS of cigarette. The removal yields for tar and nicotine were strictly dependent on the mean sizes and masses of the sepiolite granules. Therefore, various filter tips with desired removal yields can be prepared by modifying either of these parameters. Organic molecules in the MSS are retained on the fibres of the cluster structure of sepiolite granules rather than in the voids between them. However, a portion of the nicotine dissolves in the water existing in the pores of the sepiolite fibres. The crystal structure of sepiolite is not affected by the smoking process.
Financial support
Support for this work was provided by the Scientific and Technological Research Council of Turkey (TBAG-576/C) and Ankara University Research Fund (12B4240016).