INTRODUCTION
Within the European peatland deposits numerous subfossil trunks occur. Usually they are Scots pine (Pinus sylvestris L.) called bog pine, and bog oak (Quercus robur), other species are less common (e.g., Pinus cembra) (Leuschner et al. Reference Leuschner, Spurk, Baillie and Jansma2000, Reference Leuschner, Sass-Klassen, Jansma, Baillie and Spurk2002; Eckstein et al. Reference Eckstein, Leuschner and Bauerochse2008; Nicolussi et al. Reference Nicolussi, Kaufmann, Melvin, van der Plicht, Schießling and Thurner2009; Achterberg et al. Reference Achterberg, Frechen, Bauerochse, Eckstein and Leuschner2017; Edvardsson et al. Reference Edvardsson, Stoffel, Corona, Bragazza, Leuschner, Charman and Helama2016b). Frequently, when subfossil trees are exposed during the exploitation of peat, they constitute a valuable material for dendrochronological analyzes (e.g., Leuschner et al. Reference Leuschner, Spurk, Baillie and Jansma2000, Reference Leuschner, Sass-Klassen, Jansma, Baillie and Spurk2002, Reference Leuschner, Bauerochse and Metzler2007; Eckstein et al. Reference Eckstein, Leuschner and Bauerochse2008; Edvardsson et al. Reference Edvardsson, Stoffel, Corona, Bragazza, Leuschner, Charman and Helama2016b; Krąpiec et al. Reference Krąpiec, Margielewski, Korzeń, Szychowska-Krąpiec, Nalepka and Łajczak2016). These types of analyses, as well as multiproxy studies of the peatland deposits in which trunks occur at different levels, allow for the reconstruction of palaeoenvironmental changes (especially climatic) during the Holocene (Gunnarson et al. Reference Gunnarson, Borgmark and Wastegārd2003; Eckstein et al. Reference Eckstein, Leuschner and Bauerochse2011; Edvardsson et al. Reference Edvardsson, Stoffel, Corona, Bragazza, Leuschner, Charman and Helama2016b; Achterberg et al. Reference Achterberg, Eckstein, Birkholz, Bauerochse and Leuschner2018).
Up to now, the numerous dendrochronological studies of pine and oak trees have been carried out in peatlands of Germany (Leuschner et al. Reference Leuschner, Sass-Klassen, Jansma, Baillie and Spurk2002, Reference Leuschner, Bauerochse and Metzler2007; Eckstein et al. Reference Eckstein, Leuschner and Bauerochse2008, Reference Eckstein, Leuschner, Giesecke, Shumilovskikh and Bauerochse2010, Reference Eckstein, Leuschner and Bauerochse2011; Achterberg et al. Reference Achterberg, Frechen, Bauerochse, Eckstein and Leuschner2017, Reference Achterberg, Eckstein, Birkholz, Bauerochse and Leuschner2018), England (Lageard et al. Reference Lageard, Chambers and Thomas1999, Reference Lageard, Thomas and Chambers2000), Scotland (Moir et al. Reference Moir, Leroy, Brown and Collins2010; Moir Reference Moir2012), Ireland (Pilcher et al. Reference Pilcher, Baillie, Brown, McCormac, MacSweeney and McLawrence1995; Torbenson et al. Reference Torbenson, Plunkett, Brown, Pilcher and Leuschner2015), Sweden (Gunnarson Reference Gunnarson1999; Gunnarson et al. Reference Gunnarson, Borgmark and Wastegārd2003, Reference Gunnarson2008; Edvardsson Reference Edvardsson2010; Edvardsson et al. Reference Edvardsson, Leuschner, Linderson, Linderholm and Hammarlund2012a, Reference Edvardsson, Linderson, Rundgren and Hammarlund2012b), Finland (Helama et al. Reference Helama, Lindholm, Timonen and Eronen2004, Reference Helama, Kuoppama and Sutinen2020), and Lithuania (Pukienè Reference Pukienè2001; Vitas Reference Vitas2009; Edvardsson et al. Reference Edvardsson, Corona, Mazeika, Pukienè and Stoffel2016a) (Figure 1A—sites: 1, 3–5, 7–9). Bog oak chronologies are known from peatlands of the Netherlands (Jansma Reference Jansma1996; Sass-Klassen and Hanraets Reference Sass-Klassen and Hanraets2006) and Denmark (Edvardsson et al. Reference Edvardsson, Corona, Mazeika, Pukienè and Stoffel2016a) (Figure 1A—sites: 2 and 6). Such studies were also carried out in the Alps based on the Stone pine (Pinus cembra) (Figure 1A—site 10) (Nicolussi et al. Reference Nicolussi, Kaufmann, Patzelt, van der Plicht and Thurner2005, Reference Nicolussi, Kaufmann, Melvin, van der Plicht, Schießling and Thurner2009) and (based on subfossil Pinus and Spruce) in Romania (the Carpathians) (Figure 1A—site 11) (Árvai et al. Reference Árvai, Popa, Mîndrescu, Nagy and Kern2016).

Figure 1 Study area: A—a location of the Podemszczyzna peatland, compared to the location of the other European peatlands with Scots pine (Pinus sylvestris); B—LiDAR DTM of the Podemszczyzna fen. C—peat extraction by excavator; D—post-exploitation ponds in the Podemszczyzna fen. Photos by W. Margielewski. On the section A, sites with dendrochronologically analyzed subfossil pine bogs (Pinus sylvestris) and oak (Quercus robur) are marked: 1—Germany (Leuschner et al. Reference Leuschner, Bauerochse and Metzler2007; Eckstein et al. Reference Eckstein, Leuschner and Bauerochse2011; Achterberg et al. Reference Achterberg, Eckstein, Birkholz, Bauerochse and Leuschner2018); 2—the Netherlands (Jansma Reference Jansma1996; Sass-Klassen and Hanraets Reference Sass-Klassen and Hanraets2006); 3—England (Lageard et al. Reference Lageard, Chambers and Thomas1999, Reference Lageard, Thomas and Chambers2000); 4—Scotland (Moir et al. Reference Moir, Leroy, Brown and Collins2010; Moir Reference Moir2012); 5—Ireland (Pilcher et al. Reference Pilcher, Baillie, Brown, McCormac, MacSweeney and McLawrence1995; Torbenson et al. Reference Torbenson, Plunkett, Brown, Pilcher and Leuschner2015); 6—Denmark (Edvardsson et al. Reference Edvardsson, Stoffel, Corona, Bragazza, Leuschner, Charman and Helama2016b); 7—Sweden (Edvardsson Reference Edvardsson2010; Edvardsson et al. Reference Edvardsson, Leuschner, Linderson, Linderholm and Hammarlund2012a, Reference Edvardsson, Linderson, Rundgren and Hammarlund2012b); 8—Finland (Helama et al. Reference Helama, Lindholm, Timonen and Eronen2004, Reference Helama, Kuoppama and Sutinen2020); 9—Lithuania (Pukienè Reference Pukienè2001; Edvardsson et al. Reference Edvardsson, Corona, Mazeika, Pukienè and Stoffel2016a); 10—Alps (Pinus cembra) (Nicolussi et al. Reference Nicolussi, Kaufmann, Patzelt, van der Plicht and Thurner2005, Reference Nicolussi, Kaufmann, Melvin, van der Plicht, Schießling and Thurner2009); 11—Southern Carpathians (stone Pine) (Árvai et al. Reference Árvai, Popa, Mîndrescu, Nagy and Kern2016). Sites in Polish territory (bog pines and oak): 12—Rucianka (Barniak et al. Reference Barniak, Krąpiec and Jurys2014); 13—Imszar (Margielewski et al. Reference Margielewski, Krąpiec, Kupryjanowicz, Fiłoc, Buczek, Stachowicz-Rybka, Obidowicz, Pociecha, Szychowska-Krąpiec, Sala and Klimek2022a); 14—Puścizna Wielka (Krąpiec et al. Reference Krąpiec, Margielewski, Korzeń, Szychowska-Krąpiec, Nalepka and Łajczak2016); 15—Podemszczyzna (this study). Other sites in Poland marked on the map—under development.
In the Polish territory dendrochronological studies of bog pine and bog oak trees have been so far conducted for the Rucianka peatland (Polish Lowland) (Figure 1A—site 12) (Barniak et al. Reference Barniak, Krąpiec and Jurys2014). Bog pine chronology was also studied in the Imszar raised bog (Polish Lowland) (Figure 1A—site 13) (Margielewski et al. Reference Margielewski, Krąpiec, Kupryjanowicz, Fiłoc, Buczek, Stachowicz-Rybka, Obidowicz, Pociecha, Szychowska-Krąpiec, Sala and Klimek2022a) and in the Puścizna Wielka raised bog (Polish Carpathians, southern Poland—see Figure 1A—site 14) (Krąpiec and Szychowska-Krąpiec Reference Krąpiec and Szychowska-Krąpiec2016; Krąpiec et al. Reference Krąpiec, Margielewski, Korzeń, Szychowska-Krąpiec, Nalepka and Łajczak2016). The newest site, where subfossil trunks were excavated during the peat exploitation, is the Podemszczyzna peatland situated in Southeastern Poland (Figure 1A—site 15). In this case, bog pine and deciduous trees dendrochronology, along with peat analysis using pollen, non-pollen palynomorphs, Cladocera, geochemical and lithological analyses, provided the basis for the examination of the influence of climate on the phases of growth and dying off of trees in the peatland area.
THE STUDY AREA
Podemszczyzna peatland is located in the village of Podemszczyzna, in the SE part of the Tarnogród Plateau, which is part of the Sandomierz Basin, in close proximity to the Roztocze Upland (Figure 1A) (Kondracki Reference Kondracki2001). A fen, with an area of 25 ha, was formed in a depression developed within the Świdnica river valley which contemporary flows in the vicinity (Figure 1B). Nowadays, peat is being exploited here (with the use of peat excavator) for balneological purposes of the nearby Horyniec health resort (Figure 1C). Two artificial ponds were formed in post-exploitation depressions (Figure 1 C–D). During the peat extraction, several dozen pieces of wood were excavated, including numerous subfossil tree trunks which were used for dendrochronological analysis (Figure 2).
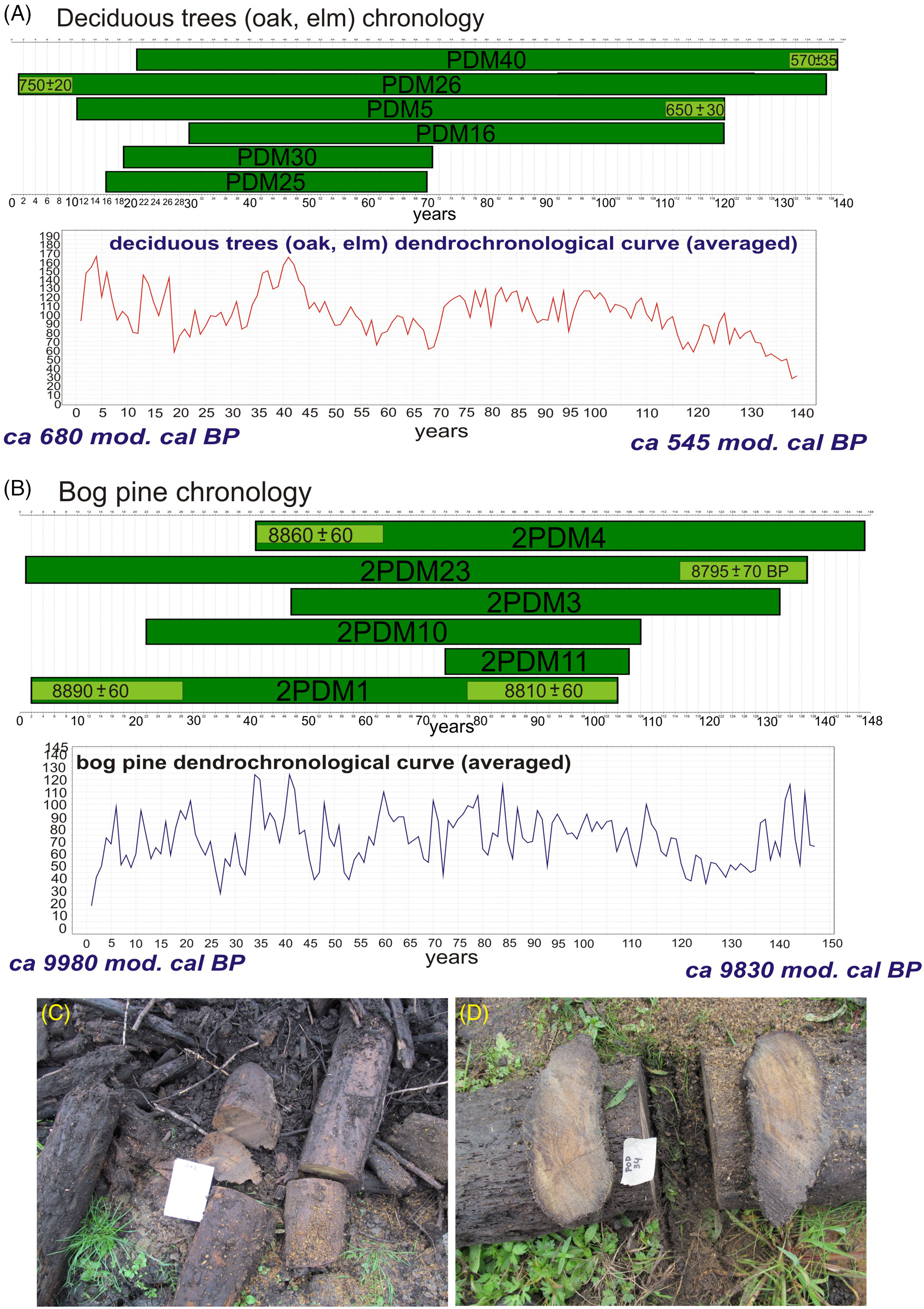
Figure 2 The subfossil trees floating chronologies (with time ranges) and dendrochronological curves of the Podemszczyzna fen on the base of wiggle-matching: A—deciduous trees (oak, elm) chronology; B—bog pine chronology; C and D—subfossil trees (sampled) in Podemszczyzna fen, extracted during peat exploitation. Photo by W. Margielewski.
MATERIALS AND METHODS
Coring and Sampling
Coring was performed in the central parts of the peatland, in the area between two post-exploitation ponds (Figure 1B) (GPS coordinates 50º12.768'N, 23º17.861'E). Drilling was performed using an Instorf sampler, 10 cm in diameter. Two complete logs of peat were taken for multiproxy analysis.
Samples for dendrochronological analysis were collected from subfossil tree trunks extracted during the peat exploitation, using a gas-engine chainsaw (Figure 2C and D). In total, 40 samples of wood (wood slices) of various species were collected.
Sediment Analysis
Organic deposits underwent a plant tissue analysis by means of a light microscope. The peat type was determined in accordance with the classification of Tołpa et al. (Reference Tołpa, Jasnowski and Pałczyński1971) (Figure 3A). Loss on ignition (LOI) analysis of deposits were elaborated for each 2.5 cm sequence of log. The samples were subjected to ignition in a muffle furnace at 550ºC according to the procedure of Heiri et al. (Reference Heiri, Lotter and Lemcke2001).
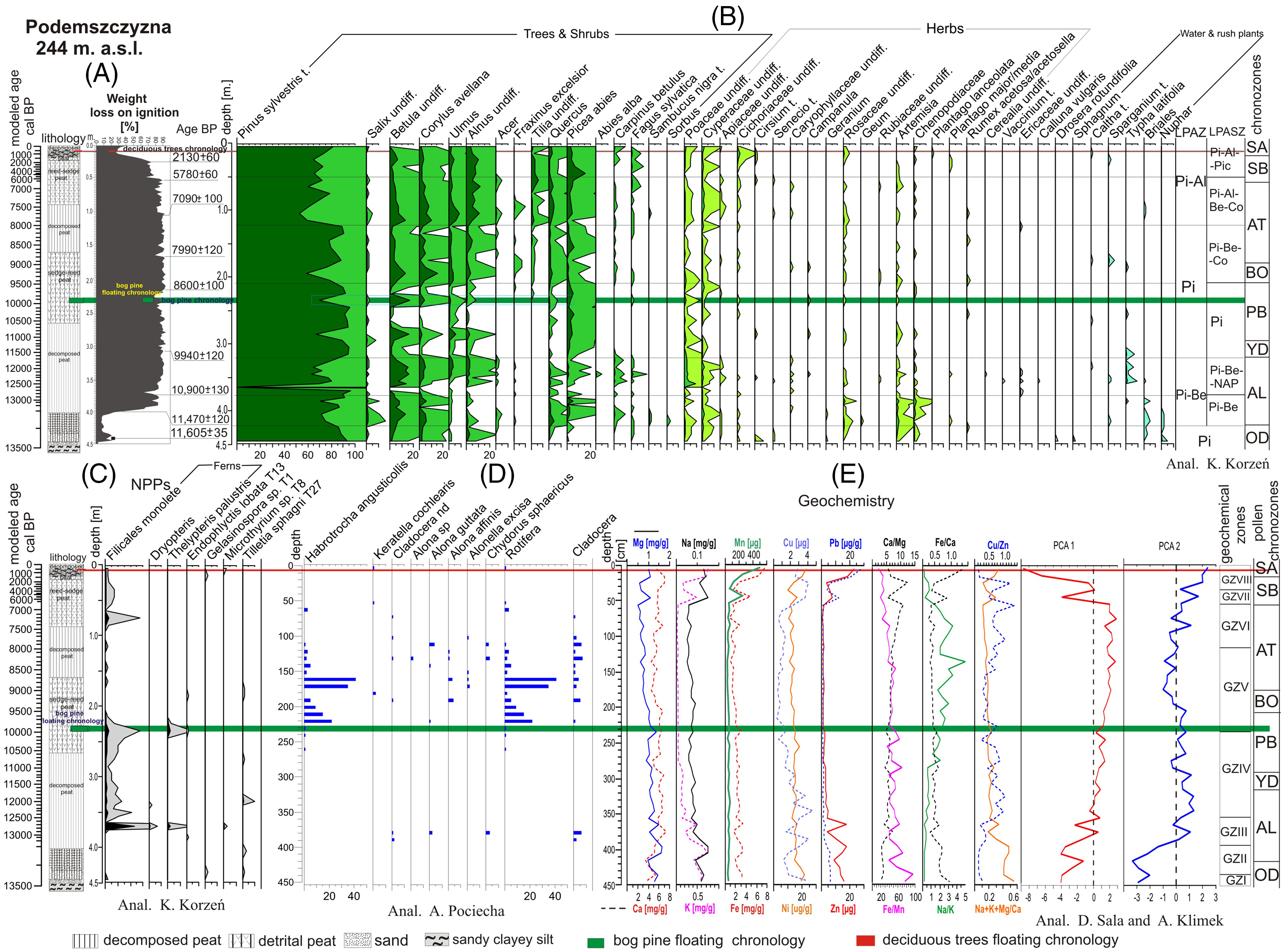
Figure 3 A—Litostratigraphic sequence of the Podemszczyzna fen with loss on ignition curve (A) and with modeled age scale (on the basis of age-depth model). The position of subfossil trees in the deposits was reconstructed on the basis of modeled age. B—pollen percentage diagram of terrestrial plants (selected taxa), with LPAZ and chronozones (det. by K. Korzeń); C—percentage diagram of aquatic, mire plants, and non-pollen palynomorphs (NPPs) (det. by K. Korzeń), D—percentage diagram of Rotifera and Cladocera (det. by A. Pociecha), E—geochemistry of peatland deposits (det. by D. Sala and A. Klimek). PCA1 and PCA2 calculated by K. Buczek.
Dendrochronological Analysis
Tree species were identified anatomically (see Schweingruber Reference Schweingruber1988, Reference Schweingruber1990; Godet Reference Godet2008). Annual tree rings were precisely measured (with the accuracy of 0.01 mm), using the Dendrolab 1.0 equipment (Zielski and Krąpiec Reference Zielski and Krąpiec2004). Processing of the measured tree-ring sequences was carried out using the TREE-RINGS software (Krawczyk and Krąpiec Reference Krawczyk and Krąpiec1995), and the TSAP computer programme (Rinn Reference Rinn2005).
The crossdates of samples were statistically validated, using t-coefficient (Baillie and Pilcher Reference Baillie and Pilcher1973), and “Gl coefficient” (Gleichlaeufigkeit; Eckstein and Bauch Reference Eckstein and Bauch1969). The accuracy of the measurements and the quality of the cross-dating were verified by the COFECHA software (Holmes Reference Holmes1999).
The relative age of wood samples, enabling the development of floating chronologies, have been determined by the wiggle-matching method with a use of the OxCal computer program, v. 4.3 (Bronk Ramsey Reference Bronk Ramsey2009) (Figure 2A and B).
Radiocarbon Dating
Conventional radiocarbon dating of organic material (peat, wood) using the LSC (liquid scintillation counting) method was conducted in the Laboratory of Absolute Dating in Kraków, Poland, according to standard procedure (Skripkin and Kovalyukh Reference Skripkin and Kovalyukh1998). AMS dating was made in the Laboratory of Absolute Dating in Kraków, Poland (graphite preparation) and in the University of Georgia, USA (measurements) (Cherkinsky et al. Reference Cherkinsky, Culp, Dvoracek and Noakes2010).
Ten 14C dates of organic deposits and seven dates of wood (enabling wiggle-matching analysis) were obtained (Table 1). Calibrated radiocarbon dates (denoted by “cal BP” abbreviation) with 95.4% probability, were determined using the OxCal computer program v. 4.3 (Bronk Ramsey Reference Bronk Ramsey2009, Reference Bronk Ramsey2017) and the IntCal20 radiocarbon calibration dataset (Reimer et al. Reference Reimer, Austin, Bard, Bayliss, Blackwell, Bronk Ramsey, Butzin, Cheng, Edwards, Friedrich, Grootes, Guilderson, Hajdas, Heaton, Hogg, Hughen, Kromer, Manning, Muscheler, Palmer, Pearson, van der Plicht, Reimer, Richards, Scott, Southon, Turney, Wacker, Adolphi, Büntgen, Capano, Fahrn, Fogtmann-Schulz, Friedrich, Köhler, Kudsk, Miyake, Olsen, Reinig, Sakamoto, Sookdeo and Talamo2020).
Table 1 14C dates of samples taken from the log cored in the Podemszczyzna peatland and wood fragments used for wiggle-matching analysis.

The age-depth model was elaborated using the OxCal computer program (v. 4.4.3), and their P_sequence function with the following parameters: k0= 0.8, log10(k/k0) = 1 and interpolation = 0.5 cm (Bronk Ramsey Reference Bronk Ramsey2009), based on IntCal 20 dataset (Reimer et al. Reference Reimer, Austin, Bard, Bayliss, Blackwell, Bronk Ramsey, Butzin, Cheng, Edwards, Friedrich, Grootes, Guilderson, Hajdas, Heaton, Hogg, Hughen, Kromer, Manning, Muscheler, Palmer, Pearson, van der Plicht, Reimer, Richards, Scott, Southon, Turney, Wacker, Adolphi, Büntgen, Capano, Fahrn, Fogtmann-Schulz, Friedrich, Köhler, Kudsk, Miyake, Olsen, Reinig, Sakamoto, Sookdeo and Talamo2020). Sections of log, where the changes in accumulation rate are expected, were marked using Boundary command (Bronk Ramsey Reference Bronk Ramsey2009). As a result, a modeled age (mod. cal BP) for each 0.5 cm sequence of deposits was obtained.
Pollen and Non-Pollen Palynomorphs (NPPs) Analyses
Samples of 1 cm3 in volume were prepared using the standard procedure (Berglund and Ralska-Jasiewiczowa Reference Berglund, Ralska-Jasiewiczowa and Berglund1986; Moore et al. Reference Moore, Webb and Collinson1991). Pollen analysis of 60 samples (taken for each 5–10 cm) was performed (Figure 3B). Pollen analysis was carried out with an Olympus BX43 light microscope with a magnification of 600×. More than 1000 terrestrial pollen grains were counted in each sample. NPPs were analyzed along with the pollen (Figure 1C), according to van Geel (Reference van Geel1978, Reference van Geel, Smol, Birks and Last2001), Bell (Reference Bell1983), Jankovská and Komárek (Reference Jankowská and Komárek2000) procedures.
Calculations and presentation of pollen and NPPs data were performed using TILIA Graph software (Grimm Reference Grimm1991). The pollen diagram of terrestrial taxa (Figure 3B) was divided into local pollen assemblage zones (LPAZ) and local pollen assemblage subzones (LPASZ) (Figure 1B). Aquatic pollen and non-pollen palynomorphs are shown in a separate diagram (Figure 3C)
Cladocera and Rotifera
Samples (of 1 cm3 in volume) taken for each 10 cm of the profile, were prepared according to procedure proposed by Frey (Reference Frey and Berglund1986, Reference Frey1987). The extracted Cladocera and Rotifer remains, were stored in 10 mL of water with glycerine and safranine. Taxa were identified and counted at 200 or 400× magnification under a Nikon 50i microscope. All skeletal parts were counted: headshields, shells, postabdomens and postabdominal claws. The results of analyses are presented in diagram elaborated using TILIA Graph computer program (Grimm Reference Grimm1991) (Figure 3D).
Geochemistry
Geochemical analysis was performed for 45 samples taken for each 10 cm of the profile. Samples were prepared according to the procedure proposed by Pansu and Gautheyrou (Reference Pansu and Gautheyrou2006). The content of macroelements (Ca, Mg, Na, K, Fe) and trace elements (Mn, Cu, Zn, Ni, Pb) were measured using Atomic Absorption Spectrometry (AAS) with a Thermo Scientific iCE 3500 apparatus. On the geochemical diagram developed in TILIA Graph computer program (Grimm Reference Grimm1991), were presented both: the variability of the content of the main elements in the profile (Mg, Na, Mn, Cu, Pb), and geochemical environment indicators: Na/K, Ca/Mg, Na+K+Mg/Ca, Fe/Mn, Fe/Ca Cu/Zn (Figure 3E). Based on the contents of 11 geochemical elements, the Principal Component Analysis (PCA) was carried out using Statistica 13 and its results were presented on the diagram (Figure 3E).
RESULTS AND INTERPRETATION
Sedimentary Sequence
In the bottom parts of the analyzed sediment profile, in the interval of 4.5–4.0 m, sandy silt occurs. The accumulation of organic sediments begins at a depth of approx. 4 m. It is represented by strongly decomposed peat (decomposition degree R> 65%), without mineral deposit admixture. The sludge is characterized by high losses on ignition (90%) (Figure 3A). Higher in the sediment profile, in the interval of 2.5–1.6 m, there is a sedge peat (containing Carex sp.), with admixture of common reed (Phragmites australis) macro-remnants. In the interval of 1.6–0.9 m, strongly decomposed sedge peat (R> 65%) appears again, and above it (in the interval of 0.9–0.2 m) sedge-reed peat (Magnocaricioni) was accumulated. In the upper parts of the peat complex (the last 20 cm), occurring peat is again heavily decomposed (R> 65%) and contains a large admixture of mineral sediment. The deposit is characterized by a high ash content (only 25% loss on ignition) (Figure 3A).
Dendrochronology
Among 40 samples taken during field works, 31 samples of subfossil wood were used for dendrochronological analysis (8 samples of pine trees, and 23 samples of deciduous trees). The other samples could not be analyzed due to the poor condition of the wood or too few annual rings. The average age of analyzed trees was 83 years for pines, and 87 for deciduous trees, whereas the age of individual trees ranged from 30–50 to a maximum of 137 years (Figure 2A, B).
Cross-analysis (crossdates) of all samples, analyzed separately for Pinus and deciduous trees, allowed to identify onest showing the greatest similarity. Based on the best correlated dendrochrochonological sequences, the mean dendrochronological curve of Pinus sylvestris lasting 147 years was determined (Figure 2B). The chronology is confirmed by high mean values of t-coefficient (6.6) and Gl being 70.5% (P<0.01). The second, younger chronology was compiled on the basis of deciduous trees samples (Oak, Elm) and is 139 years long (t=8.3; Gl=77%) (Figure 2A).
Relative age of the both floating chronologies was obtained using the wiggle-matching technique. The tree rings selected for the radiocarbon dating and wiggle-matching analyses and results are presented in Figure 2A and B, and in Table 1. Based on wiggle-matching analysis, the time range of the floating pine chronology was determined at ca. 9980–9830 mod. cal BP (Figure 2B). In turn, the time span of the younger floating chronology of deciduous trees is ca. 680–545 mod. cal BP (Figure 2A).
Local Vegetation History
Local vegetation history was compiled for individual chronozones based on the characteristics of the Local Pollen Assemblages Zones (LPAZ) and Local Pollen Assemblages Subzones (LPASZ) (Figure 3B). Absolute age of pollen zones limits was determined on the basis of the modeled age (mod. cal BP).
Older Dryas Stadial (Pinus LPAZ) (before 13,386 mod. cal BP)
During this period, the dominance of pine forests and the local, very small share of alder (Alnus sp.) is visible. The share of herbaceous plants is marginal, limited mainly to grasses (Poaceae), and sedges (Cyperaceae) and mugwort (Artemisia). There is also some pollen of aquatic plants (Nuphar).
Allerød Interstadial (Pinus–Betula LPAZ) (13,386–11,584 mod. cal BP). The Allerød Interstadial here is clearly bipartite (Figure 3B). In the older part (AL1) (Pinus-Betula LPASZ) the stand is dominated by Pinus sylvestris and Betula, sometimes with an admixture of Picea and thermophilous species: Quercus, Carpinus and Corylus. Salix and Alnus occur locally. Artemisia and Chenopodiaceae have a large share among the herbaceous vegetation. The presence of grass pollen (Poaceae) and sedges (Cyperaceae) is also noted. In the younger part of Allerød Interstadial (AL2) (LPASZ: Pinus-Betula-NAP), pine (Pinus), and birch (Betula) are still dominant, but the share of other woody species, including those with higher thermal requirements (Corylus, Ulmus, Quercus, Carpinus) is significant. Picea, Fagus and (traces of) Abies alba also appear. A similar share of pollen of thermophilous plants in the Late Glacial deposits, accompanied by pollen of Fagus and Abies, was stated by K. Mamakowa (Reference Mamakowa1962) in the Allerød sedimentary sequences of the Sandomierz Basin peatlands (Podbukowina, Świlcza, Obary peatlands), linking their occurrence with a presence of on-site refugia, rather than with reworking of pollen grains from older sediments or with a long transport (Mamakowa Reference Mamakowa1962). The share of Poaceae and Cyperaceae communities is increasing, and there are also Artemisia and Chenopodiaceae.
Younger Dryas Stadial . Pinus LPASZ (11,584–11,061 mod. cal BP). The forest communities were dominated by Pinus (which is characterized by expansion here) with an admixture of Picea. The cooling of the climate was clearly visible in the form of a drastic decrease in the share of most trees, including Betula, Alnus, and especially thermophilous species (Corylus, Ulmus, Quercus).
Preboreal Phase . Pinus LPASZ (11,061–9448 mod. cal BP). In general, during the Preboreal Phase, two episodes in the initial and final period of this chronozone are clearly visible, related to the reduction of the pine’s range and the short-term expansion of Betula, Alnus and thermophilous Corylus, Ulmus and Quercus. These two “warm” episodes are separated by a clear, sharp decline of a percentage of the above-described taxa, probably related to the cooling of the Preboreal Oscillation (Björck et al. Reference Björck, Rundgrend, Ingolfssen and Vunder1997). In the youngest part of the Preboreal Phase, Tilia and Fagus appear, and there is also a greater proportion of ferns (Filicales monolete and Thelypteris palustris) (Figure 3C). Herbaceous plants are relatively poorly represented in this period, mainly by Poaceae and Cyperaceae.
Boreal Phase. Pinus-Betula-Corylus LPASZ (9448-6871 mod. cal BP). The decrease in the proportion of pine pollen is accompanied by an increase (sometimes rapid) in the proportion of Betula, Alnus, Corylus, Ulmus, Fraxinus, Tilia, Quercus, as well as a small share of Carpinus and Fagus–pollen of these last two taxa was already found in Boreal sedimentary sequence of the Sandomierz Basin peatlands (Mamakowa Reference Mamakowa1962). A gradual transformation of forests is taking place. Pine forests are partially replaced by mixed forests with deciduous trees. The herbaceous vegetation is represented by Poaceae and Cyperaceae.
Atlantic Phase (8983–6871 mod. cal BP). The Atlantic Phase is clearly bipartite. In the lower part (Picea-Betula-Corylus LPASZ), a gradual decrease in the range of mixed forests with the simultaneous expansion of Pinus and an increase in the share of Picea. The appearance of pollen from aquatic plants (Caltha, Typha, Sparganium) may indicate that the bog was flooded with water and the climate humidity growth occurred at that time. Since the middle part of the Atlantic Phase (Picea-Alnus-Betula-Corylus LPASZ) a renewed regression of Pinus and an increase in the share of deciduous trees in forest communities is observed, with a significant share of Quercus. The forest communities are represented by mixed forests. The Alnus expansion indicates the appearance of alder forest. There is a marked increase in the share of herbaceous plants (Poaceae, Cyperaceae).
Subboreal Phase. During the older part of Pinus-Alnus-Picea LPASZ (6871–1423 mod. cal BP), the woodlands are still dominated by pine forests, with a large share of Picea and a smaller share of deciduous trees. There is a clear regression of thermophilous species: Corylus, Ulmus, Quercus, Tilia. Picea achieves the maximum proportion of pollen in the entire profile. The share of Fagus sylvatica pollen is increasing. Locally occurring alders (high share of Alnus pollen) are quite distinct. Herbaceous plants are weakly represented in the pollen spectrum.
Subatlantic Phase. Younger part of Pinus-Alnus-Picea LPASZ (1423 mod. cal BP–present day). The woodlands are still dominated by thinned Pinus forests with a small admixture of deciduous trees, the regression of which is clearly visible in the diagram. There is a significant increase in the share of herbaceous plants (Poaceae, Cyperaceae, Cichoriaceae) in plant assemblages.
Characteristic for the palynological profile of the peat bog are frequent fluctuations in the proportion of pine pollen in the diagram with the general dominance of pollen of this species. The local decrease in the share of pine is always accompanied by an increase in the share of the Betula pollen, as well as pollen of the thermophilous taxa: Corylus, Ulmus, Alnus, and Quercus (Figure 3B).
Rotifera and Cladocera
In the Podemszczyzna sedimentary sequence, presence of 7 taxa belonging to two systematic groups has been confirmed: rotifers (2 taxa) and cladocerans (5 taxa) (Figure 3D). In samples containing Cladocera it was, however, impossible to identify them to a lower systematic degree. Their chitinous remains were very damaged and very thin.
Among rotifers taxa two species were recognized: first belonging to Bdelloidea: Habrotrocha angusticollis and second belonging to Monogononta: Keratella cochlearis. Shells of H. angusticollis were found in significant densities in the sediment samples corresponding to the end part of the Preboreal Phase (PB) (Figure 3D). The highest densities of these species were identified at the depths of 160 cm–41 ind. cm3; 170 cm–35 ind. cm3, 210 cm–15 ind. cm3, and 220 cm–22 ind. cm3 . K. cochlerais was found in a trace amount in the samples of Atlantic and Subboreal Phases (Figure 3D).
In Cladocera group all identified taxa belonged to the Chydoridae family, which is a littoral taxa group. Due to a small density of Cladocera taxa, which ranges between 1 to 4 ind. cm3, the dominant taxa could not be distinguished. Cladocera remains were found in the Atlantic Phase (170–100 cm interval), in the Boreal Phase (190 cm), some single pieces in the Preboreal Phase (220 cm) and in the Allerød Interstadial (380–390 cm) (Figure 3D).
On the basis of taxonomic analysis and environmental preferences, two groups of organisms were distinguished: the first one related to the acidic environment and wet area with low level of water (H. angusticollis, Alonella excisa, and Chydorus sphaericus) and the second one related to the open space of stagnant water Keratella cochlearis (Figure 3D).
Geochemical Analysis
In the sedimentary profile of the Podemszczyzna peatland eight geochemical zones (GZI-GZVIII) were distinguished that differ in content of geochemical elements and indicators of the sedimentation environment (Figure 3E).
GZ I (4.45–4.35 m; 13,490–13,440 mod. cal BP). First geochemical zone is related to the deposition of sandy clayey silt underlying the peat sequence. The horizon is marked by slightly increasing content of organic matter (from 3.5 to 18.0%) and occurrence of almost all lithophilic and trace elements (except Cu). In relation to the whole profile, values of Fe/Mn and Na+K+Mg/Ca ratios reach here their maximum (95.0 and 0.56 respectively) what indicates high erosion in sedimentary basin catchment accompanied by reducing condition of sedimentary environment caused by water table rising.
GZ II (4.35–3.95 m; 13,440–13,290 mod. cal BP). This zone is characterized by oscillating but generally high concentrations of elements as well as changeable content of organic matter. Moreover, concentration of elements increases significantly reaching in case of Mg, K, Na, their highest values in the whole profile (1.60 mg/g, 0.73 mg/g, 0.15 mg/g respectively). This results in very high values of Na+K+Mg/Ca ratio (0.48) and extreme low values of Na/K (0.20). Noticeably high values of Zn and Pb in the GZ II zone are probably related to the acceleration of mechanical erosion in the sedimentary basin as well as bioaccumulation.
GZ III (3.95–3.55 m; 13,290–12,430 mod. cal BP). This geochemical zone is related to the early stage of sedge peat accumulation. Decline in concentrations of lithophilic elements, which is visible at the beginning of the phase, was associated with higher redox conditions and decreasing delivery of allochthonous material to the peatland. A noticeable increase in the contents of most of the elements (except Ca) at the depth of 3.65 m (12,650 mod. cal BP) as well as an abrupt rise of catchment erosion ratio and reducing conditions of sedimentary environment point to possible inundation of the fen by river water.
GZ IV (3.55–2.35 m; 12,430–9960 mod. cal BP). This zone is characterized by an increasing content of organic matter (up to 90%) and gradually declining content of Mg, K, Pb, Zn, and Cu. Concentration of other elements fluctuates around average values calculated for the whole profile. At the same time values of Fe/Mn ratio fluctuates reaching two distinct peaks: at 2.85 m (10,960 mod. cal BP) and 2.45–2.35 m (10,140–9960 mod. cal BP), which points to a higher water level in the peatland.
GZ V (2.35–1.35 m; 9960–8260 mod. cal BP). This geochemical zone corresponds to a period of accumulation of pure sedge peat (LOI values: 80–93%). Concentrations of elements become stable without significant fluctuations. Values of Na/K ratio increase drastically reaching the highest values (5.0) at the end of the phase which can be interpreted as higher evapotranspiration (Borówka Reference Borówka1992). Decreasing values of catchment erosion ratio (Na+K+Mg/Ca) from 0.20 to 0.15, accompanied by fairly stable low redox conditions (mean Fe/Mn: 45) points to a change in dominant denudation process from mechanical erosion to chemical denudation.
GZ VI (1.35–0.55 m; 8260–6820 mod. cal BP). This zone represents phase of sedge peat accumulation and subsequent 0.9 m sedge-reed peat accumulation. Peat is strongly decomposed (R>65%) but without significant admixture of minerogenic material (mean LOI values: 87%). This layer is marked by gradually decreasing values of Fe/Mn ratio (from 40.2 to 27.4 in the end of the phase), catchment erosion ratio (0.18–0.11) and high values of chemical denudation indicators (Na/K, Ca/Mg).
GZ VII (0.55–0.35 m; 6820–3580 mod. cal BP). Beginning of this geochemical zone is marked by a distinct increase in the concentration of all lithophilic and trace elements in oxidizing environment (mean Fe/Mn: 23.1) and substantial increasing of erosion in peatland catchment (mean Na+K+Mg/Ca: 0.19). At the same time content of organic matter (LOI) decreases from mean 87% in GZ to 72%.
GZ VIII (0.35–0 m; 3580 mod. cal BP–present). This phase is distinguished by significant fluctuations of Fe, Mn, K, Mg, Ni, Zn and Pb. Contents of these elements initially decline at the depth of 0.35 and then considerably increase, reaching in case of the Pb, Ni, Zn, Fe, and Mn their highest concentrations in the entire profile: 26.7 ug/g, 23.0 ug/g, 22.9 ug/g, 7.5 mg/g, and 506.0 ug/g respectively. Increasing values of Na+K+Mg/Ca ratio and simultaneous significant decrease of Na/K ratio indicate a change of dominant denudation process in this phase. Low values of Fe/Mn ratio suggest oxidizing conditions of sedimentary environment (decline of the water level in fen). Significantly increasing contents of Pb and Zn in this phase (51.8% and 68.2% respectively) are related to its modern atmospheric deposition caused by human impact.
Principal component analysis (PCA) was used in order to determine factors which controlled the chemical composition of the sediments. Based on concentration of 11 variables: Ca, Mg, Na, K, Fe, Mn, Cu, Ni, Zn and content of organic matter (LOI) two eigenvectors were distinguished (PCA1, PCA2) that together explain ca. 74% of total geochemical variability of the deposits. First geochemical component (PCA 1) which explains 59.7% of total geochemistry variation is directly proportional to the content of organic matter (0.76) and inversely proportional to concentrations of almost all major and trace elements (except Ca and Mg). Less important second component (PCA 2) which explain ca. 14.3% of the total geochemical variance is related mainly to content of Ca (0.85) (Figure 3E).
DISCUSSION
Radiocarbon dating and palynological analysis indicate that the sedimentary basin was formed in the Older Dryas Stadial of the Late Glacial (Figure 3A–B). The presence of pollen of aquatic plants (Nuphar, Sparganium) indicates that at that time in the sedimentary basin there was a permanent water reservoir and sedimentation was of a minerogenic character (Figure 3B). This is confirmed by geochemical indicators suggesting also significant erosion in the vicinity of the reservoir at that time (Figure 2E). The surroundings of the reservoir were dominated by pine forest with an admixture of Betula and Alnus (Figure 3B). The peat accumulation (strongly decomposed sedge peat) began during the Allerød Interstadial climate warming (Figure 3A–B). Delivery of mineral sediment to the peatland is visible as the decline of the loss on ignition curve in the middle Allerød depositional sequence (Figure 3A) and it was caused by flooding of the river waters over the fen, as geochemical indicators suggest (phase GZIII–see Figure 3E). This, in turn, may be related to the cooling and growth of climate humidity by about 13,200–12,800 cal BP known as the Gerzensee Oscillation (Amman et al. Reference Amman, Birks, Brooks, Eicher, von Grafenstein, Hofmann, Lemdahl, Schwander, Tobolski and Wick2000; Schwander Reference Schwander, Eicher and Amman2000). In the Podemszczyzna profile, the sediment record of the Allerød Interstadial is bipartite, but the usual vegetation pattern—birch dominating in the older Allerød phase and pine—in the younger phase (see Latałowa Reference Latałowa2003)–is in this case different. Two birch-pine phases are separated here by a phase with a predominance of pine (Figure 3B). In the sedimentation sequence of Allerød there is a significant share of thermophilous plants (Corylus, Ulmus, Quercus, Carpinus), which usually accompany a simultaneous decrease in the share of pine (Figure 3B). The significant share of pollen of thermophilous plants in the Allerød sedimentary sequence, which is also accompanied by Fagus and Abies, occurs within the aforementioned peatlands of the Sandomierz Basin (Mamakowa Reference Mamakowa1962). Also in the Polish Carpathians, in the Late Glacial sedimentary sequences of peatlands (Bølling Interstadial), there is sometimes a significant share of pollen of thermophilous plant taxa, invariably accompanied by Fagus and Abies (Margielewski et al. Reference Margielewski, Obidowicz, Zernitskaya and Korzeń2022b). In these cases, it would be difficult to explain the presence of such abundant share of pollen in deposits with their distant transport or redeposition. These sitesx probably constituted refugia during the Late Glacial, what was suggested by K. Mamakowa (Reference Mamakowa1962) (see also Margielewski et al. Reference Margielewski, Obidowicz, Zernitskaya and Korzeń2022b)
Climate warming in the Preboreal Phase was marked by a decrease in pine pollen concentration and an increase in a share of Betula, Alnus and thermophilous Corylus, Ulmus, Quercus (Figure 3B). In the middle part of the Preboreal Phase, however, another regression of thermophilous species is visible, the content of Picea pollen is also increasing (Figure 3B). This regression of termophilous plants dated at 10,744 ± 118–10,159 ± 114 mod. cal BP, is probably related to the cooling of the climate during the Preboreal Cold Oscillation, recorded at that time in lake sediments from Poland (Fiłoc et al. Reference Fiłoc, Kupryjanowicz, Rzodkiewicz and Suchora2016). Geochemical indicators suggest higher water level in the fen at that time (Figure 3E). In the younger phase of Preboreal, a short-term regression of pine is again noted and the sudden development of Salix, Betula, Alnus along with thermophilous Corylus, Ulmus Tilia and Quercus occurs (Figure 3B). During this period, pine trees encroachment into the fen is dated at 9980–9830 mod. cal BP (Figure 2B and 3B). The pine woodlands in the vicinity of the fen thinned out, which is indicated by the significant share of Corylus and ferns (Filicales monolete, Thelypteris palustris) (Figure 3B and C). The encroachment of pines into the fen (its surface was about 2.2 m below the modern ground level) coincided with a strong climate overdrying in Central Europe (ca. 10,200– 9600 cal BP), causing, among other phenomena, a decline of fluvial activity of the European rivers (Figure 4) (Starkel et al. Reference Starkel, Michczyńska, Krąpiec, Margielewski, Nalepka and Pazdur2013). This period was, however, not stable: the reduction of annual growth of pine indicates a local deterioration of ecological conditions ca. 9955–9945 and 9860–9845 mod. cal BP: trees dying off registered in the peat bog was associated with the latter period (Figure 2B).

Figure 4 Correlation of subfossil bog pine and bog deciduous trees (oak, elm) dendrochronology of the Podemszczyzna fen, in relation to bog pine and bog oak time range during the Holocene in other European peatlands (location of analyzed sites and authors of dendrochronological analysis—see Figure 1A), and in relation to palaeoclimatic changes in the Northern Hemisphere (Bond et al. Reference Bond, Kromer, Beer, Muscheler, Evans, Showers, Hoffman, Lotti-Bond, Hajdas and Bonani2001, Reference Bond, Evans and Muscheler2008; Starkel et al. Reference Starkel, Michczyńska, Krąpiec, Margielewski, Nalepka and Pazdur2013; Wanner et al. Reference Wanner, Mercolli, Grosjean and Ritz2015). Holocene chronostratigraphy after Mangerud et al. (Reference Mangerud, Andersen, Berglund and Donner1974), Starkel et al. (Reference Starkel, Michczyńska, Krąpiec, Margielewski, Nalepka and Pazdur2013), and Walker et al. (Reference Walker, Gibbard, Head, Berkelhammer, Björck, Cheng, Cwynar, Fisher, Gknis, Long, Lowe, Newnham, Rasmussen and Weiss2019).
Later pine trees dying off recorded in the fen was caused by the deterioration of climatic and ecological conditions: the pollen curves show a short-term regression of thermophilous species (Figure 3B). There is a sharp increase in the share of Cladocera and Rotifera in the sediments, previously unheard of, which indicates an increase in the water level in the bog (Figure 3D). In the fen sediments, just above the horizon with pine trunks, a delivery of mineral sediment to the reservoir is visible, marked as decline on the loss on ignition curve, and related to a short-term flooding of the reservoir with water (Figure 3A). The geochemical indices that show an increase in chemical denudation are also changing rapidly (Figure 3E). The cooling of the climate at that time coincided with this moistening (Figure 4). In the period after 9600 cal BP, a distinct rise of fluvial activity, landslide intensification and debris flow in the European areas were recorded (Mayewski et al. Reference Mayewski, Rohling, Stager, Karlen, Maasch, Meeker, Meyerson, Gasse, van Kreveld, Holmgren, Lee-Thorp, Rosqvist, Rack, Staubwasser, Schneider and Steig2004; Pánek et al. Reference Pánek, Smolková, Hradecký, Baroň and Šilhán2013; Starkel et al. Reference Starkel, Michczyńska, Krąpiec, Margielewski, Nalepka and Pazdur2013; Margielewski Reference Margielewski2018).
Bog pine floating chronology from the Podemszczyzna fen is currently the oldest bog pine chronology in Polish and European territory (see Barniak et al. Reference Barniak, Krąpiec and Jurys2014; Krąpiec et al. Reference Krąpiec, Margielewski, Korzeń, Szychowska-Krąpiec, Nalepka and Łajczak2016; Krąpiec and Szychowska-Krąpiec Reference Krąpiec and Szychowska-Krąpiec2016; Margielewski et al. Reference Margielewski, Krąpiec, Kupryjanowicz, Fiłoc, Buczek, Stachowicz-Rybka, Obidowicz, Pociecha, Szychowska-Krąpiec, Sala and Klimek2022a) (Figure 4). It should be mentioned that previously some short pine chronologies for the Late Glacial (Bølling-Younger Dryas) have been elaborated in the pre-Alps, German and Polish territories, but they are, however, not related to bog trees (Schaub et al. Reference Schaub, Kaiser, Frank, Büntgen, Kromer and Talamo2008; Kaiser et al. Reference Kaiser, Friedrich, Miramont, Kromer, Sgier, Schaub, Boeren, Remmle, Talamo, Guibal and Sivan2012; Krąpiec et al. Reference Krąpiec, Szychowska-Krąpiec, Barniak, Goslar, Kittel, Michczyńska, Michczyński, Piotrowska, Rakowski and Wiktorowski2020).
Since the middle of the Atlantic Phase, pollen profile shows a significant decrease in the proportion of pine: the Betula and Alnus curves and the thermophilous species are already continuous here (Corylus, Ulmus, Tilia, Quercus), which indicates a permanent reconstruction of plant communities around the reservoir (Figure 3B). At the onset of the Subatlantic Phase, a mineral cover on the peat begins to form due to the delivery of mineral sediment to the peatland (Figure 3A). There is a level of trunks of deciduous trees within it, suggesting that the fen area was settled at ca. 680–545 mod. cal BP (ca. 1270–1305 mod. cal AD) by Quercus and Ulmus, which grew on the surface of the bog stabilized by the mineral cover (Figure 2A). Forest dieback which subsequently took place here could be related to the drastic changes in the climate (cooling, dampness) at the beginning of the Little Ice Age (see Grove Reference Grove1988).
CONCLUSIONS
On the basis of dendrochronological analysis and wiggle-matching dating of subfossil tree trunks extracted during peat exploitation of the Podemszczyzna fen (SE Poland), which was formed in the Older Dryas Stadial, two short floating chronologies were elaborated: bog Pine chronology and deciduous trees chronology. Older, bog pine floating chronology is 147 years long and indicates that pine trees used to grow on the Podemszczyzna peatland area during the period spanning ca. 9980–9830 mod. cal BP. It is the oldest bog pine chronology reported in the European peatlands so far. The floating chronology of deciduous trees (Oak, Elm) is 139 years long and comprises a time range of 680–545 mod. cal BP. In turn, it is the youngest chronology of deciduous trees growing in peatlands in Europe.
Dendrochronology and peat multi-proxy analyzes indicate that the encroachment of trees into the fen area took place during climate warming, when the groundwater level was lowered and the fen was desiccated. Trees dying off was, in turn, caused by groundwater level rising which occurred during climate humidity growth in the Holocene.
ACKNOWLEDGMENTS
This study was supported with funds from the National Science Centre, Poland, grant No. 2017/25/B/ST10/02439 (2018–2022). The Authors would like to thank the Board of Health Resort Horyniec for making it possible to conduct research in the area of the Podemszczyzna peatland and for their help in the research. We would like also to thank MSc Marek Górnik, and MSc Eng. Andrzej Kalemba from the Institute of Nature Conservation, Polish Academy of Sciences for theirs help in the field work.