1. Introduction
The Tibetan Plateau shares a long and complex geological history involving multiple episodes of spreading, subduction and continental collision in the Tethys Ocean basin. The Bangong–Nujiang suture zone (BNSZ), located between the Lhasa terrane to the south and the Qiangtang terrane (QT) to the north, extends more than 2000 km across the central Tibetan Plateau (Fig. 1a). This zone is generally considered the locus of the ancient Bangong–Nujiang Ocean (BNO) (Yin & Harrison, Reference Yin and Harrison2000; Kapp et al. Reference Kapp, Yin, Manning, Harrison and Taylor2003, Reference Kapp, Yin, Harrison and Ding2005; Pan et al. Reference Pan, Ding, Yao and Wang2004; Guynn et al. Reference Guynn, Kapp, Pellen, Heizer, Gehrels and Ding2006; Zhang et al. Reference Zhang, Zhang, Tang and Xia2012; Li et al. Reference Li, Qin, Li, Xiao, Zhao and Chen2016; Zhu et al. Reference Zhu, Li, Cawood, Wang, Zhao, Liu and Wang2016). The closure of the BNO, followed by the subsequent collision between the Lhasa and Qiangtang terranes, significantly contributed to the initial growth of the Tibetan Plateau before the Indo-Asian collision (Kapp et al. Reference Kapp, DeCelles, Gehrels, Heizler and Ding2007; Shi et al. Reference Shi, Yang, Xu and Qi2008, Reference Shi, Huang and Chen2020; Zhang et al. Reference Zhang, Zhang, Tang and Xia2012, Reference Zhang, Xia, Zhang, Liu, Zeng, Li and Xu2014; Zhu et al. Reference Zhu, Li, Cawood, Wang, Zhao, Liu and Wang2016; Zeng et al. Reference Zeng, Xu, Chen, Wang, Huang, Xia and Li2021). However, intense controversies remain on the closure of the BNO, and two main versions of the final closure of the BNO have been proposed. The first hypothesis is that the BNO closed between the Late Jurassic and Early Cretaceous (ca. 160-145 Ma), mainly on the basis of the presence of an angular unconformity between the Lower Jurassic oceanic rocks (flysch deposits and ophiolites) and the Upper Jurassic–Lower Cretaceous shallow marine strata (e.g. Xu et al. Reference Xu, Schärer and Allègre1985; Dewey et al. Reference Dewey, Shackleton, Chang and Sun1988; Yin et al. Reference Yin, Xu, Liu and Li1988; Kapp et al. Reference Kapp, Yin, Manning, Harrison and Taylor2003, Reference Kapp, Yin, Harrison and Ding2005, Reference Kapp, DeCelles, Gehrels, Heizler and Ding2007; Raterman et al. Reference Raterman, Robinson and Cowgill2014). The alternative version suggests that the collision between the Lhasa terrane and QT may have occurred after the Early Cretaceous (Ca. 120-100 Ma), according to abundant Early Cretaceous oceanic island basalt (OIB)-type rocks that are overlain by or interbedded with radiolarian-bearing chert and/or limestone, the existence of a mid-Cretaceous magmatic arc in the southern QT and palaeomagnetic data (e.g. Zhu et al. Reference Zhu, Pan, Mo, Wang, Zhao and Liao2006; Bao et al. Reference Bao, Xiao, Su and Wang2007; Baxter et al. Reference Baxter, Aitchison and Zyabrev2009; Zhang et al. Reference Zhang, Zhang, Tang and Xia2012, Reference Zhang, Xia, Zhang, Liu, Zeng, Li and Xu2014; Xu et al. Reference Xu, Li, Xu, Wu, Fan and Wu2015; Chen et al. Reference Chen, Hu, Zhong, Fu, Li and Wang2018; Fan et al. Reference Fan, Li, Wang and Xie2018; Luo et al. Reference Luo, Fan, Hao, Li and Zhang2020; Shi et al. Reference Shi, Huang and Chen2020).

Figure 1. (a) Tectonic framework of the Tibetan Plateau (modified from Zhu et al. Reference Zhu, Zhao, Pan, Lee, Kang, Liao, Wang, Li, Dong and Liu2009, Reference Zhu, Li, Cawood, Wang, Zhao, Liu and Wang2016; Sui et al. Reference Sui, Wang, Zhu, Zhao, Chen, Santosh, Hu, Yuan and Mo2013); (b) simplified geologic map showing outcrops of magmatic rocks in the RCA, western Qiangtang, central Tibet [modified from Kapp et al. (Reference Kapp, Yin, Harrison and Ding2005) and Song et al. (Reference Song, Chen, Wei, Ma, Sun and Liu2021)]. Age data in this paper are in red font; other literature age data are from Chang et al. (Reference Chang, Zhu, Zhao, Dong, Mo, Liu and Hu2011), Kapp et al. (Reference Kapp, Yin, Harrison and Ding2005), Hao et al. (Reference Hao, Wang, Wyman, Ou, Dan, Jiang, Wu, Yang, Long and Li2016 a) and Song et al. (Reference Song, Chen, Wei, Ma, Sun and Liu2021).
The Rena-Co area (RCA) is located in the southern margin of the QT to the north of the BNSZ (Fig. 1b). Late Mesozoic volcanic rocks are widely distributed within this area (e.g. Kapp et al. Reference Kapp, Yin, Harrison and Ding2005; Chang et al. Reference Chang, Zhu, Zhao, Dong, Mo, Liu and Hu2011; Li et al. Reference Li, Qin, Li, Xiao, Zhao and Chen2016; Wei et al. Reference Wei, Tang, Song, Liu, Feng and Li2017), and this area has been a research focus in recent years (Kapp et al. Reference Kapp, Yin, Harrison and Ding2005; Chang et al. Reference Chang, Zhu, Zhao, Dong, Mo, Liu and Hu2011; Li et al. Reference Li, Qin, Li, Richards, Zhao and Cao2014 a; Hao et al. Reference Hao, Wang, Wyman, Ou, Dan, Jiang, Wu, Yang, Long and Li2016 a, b; Song et al. Reference Song, Chen, Wei, Ma, Sun and Liu2021). It is well accepted that understanding Cretaceous volcanism in the southern margin of the QT is of great significance for understanding the evolution of the BNO and Qiangtang–Lhasa collisional orogeny (Yin & Harrison, Reference Yin and Harrison2000; Kapp et al. Reference Kapp, Yin, Manning, Harrison and Taylor2003, Reference Kapp, Yin, Harrison and Ding2005; Zhu et al. Reference Zhu, Li, Cawood, Wang, Zhao, Liu and Wang2016). Late Mesozoic volcanic rocks, especially the dacites of the Meiriqiecuo Formation (MF) from the RCA, could play an important role in constraining the tectonic setting at that time. However, the detailed petrogenesis of the Early Cretaceous dacites in the RCA remains unknown.
In this paper, we focus on the MF dacites from the RCA in the southern QT, central Tibet. Here, we present new zircon U-Pb ages, whole-rock major and trace element compositions, and whole-rock Sr-Nd-Pb and in situ zircon Hf isotope data for the RCA dacites. Based on our new data, we provide a new tectono-magmatic model to explain the Early Cretaceous magmatism in the southern QT and constrain the petrogenetic processes of the RCA dacites and the time of the closure of the BNO.
2. Geological background and petrographic observations
The Tibetan Plateau is composed of four continental terranes from south to north: the Himalaya, Lhasa, Qiangtang, and Songpan–Ganzi. These terranes are separated by the Indus–Yarlung Zangbo suture zone (IYZSZ), Bangong–Nujiang suture zone (BNSZ), and Jinshajiang suture zone (JSSZ) (Yin & Harrison, Reference Yin and Harrison2000; Shi et al. Reference Shi, Yang, Xu and Qi2008; Zhu et al. Reference Zhu, Li, Cawood, Wang, Zhao, Liu and Wang2016). The BNSZ is 20–95 km wide in a N-S direction and >2200 km long in an E-W direction and represents a geologically significant suture zone that stretches from Bangongco to the west via Gerze, Nyima, Dongqiao, Amdo and Dêngqên to the east and then turns south into the Mogok area of Myanmar (Yin & Harrison, Reference Yin and Harrison2000; Kapp et al. Reference Kapp, Yin, Manning, Harrison and Taylor2003, Reference Kapp, Yin, Harrison and Ding2005). As the boundary of the LT and the QT, the BNSZ contains abundant Jurassic-Cretaceous flysch, mélange and scattered ophiolitic fragments (Sengör, Reference Sengör1979; Girardeau et al. Reference Girardeau, Marcoux, Allegre, Bassoullet, Tang, Xiao, Zao and Wang1984; Yin et al. Reference Yin, Xu, Liu and Li1988; Kapp et al. Reference Kapp, Yin, Manning, Harrison and Taylor2003). To the north of the BNSZ, the southern QT is composed of Carboniferous–Permian shelf strata along with Jurassic marine rocks exposed along its northern and southern margins (Girardeau et al. Reference Girardeau, Marcoux, Allegre, Bassoullet, Tang, Xiao, Zao and Wang1984; Yin & Harrison, Reference Yin and Harrison2000; Pan et al. Reference Pan, Ding, Yao and Wang2004; Zhang et al. Reference Zhang, Zhang, Tang and Xia2012). Moreover, many late Mesozoic intermediate–felsic magmatic rocks with ages ranging from 183 to 101 Ma are widely distributed in the southern QT (e.g. Kapp et al. Reference Kapp, Yin, Harrison and Ding2005; Chang et al. Reference Chang, Zhu, Zhao, Dong, Mo, Liu and Hu2011; Li et al. Reference Li, Qin, Li, Richards, Zhao and Cao2014 a, b, Reference Li, Qin, Li, Xiao, Zhao and Chen2016; Hao et al. Reference Hao, Wang, Wyman, Ou, Dan, Jiang, Wu, Yang, Long and Li2016 a, b; Zhu et al. Reference Zhu, Li, Cawood, Wang, Zhao, Liu and Wang2016; Wei et al. Reference Wei, Tang, Song, Liu, Feng and Li2017, Reference Wei, Song, Tang, Liu, Wang, Lin, Feng, Hou and Danzhen2018; Song et al. Reference Song, Chen, Wei, Ma, Sun and Liu2021).
The study area in this paper, the Rena-Co area (RCA), is located approximately 50 km northeast of Gerze County, Tibet, on the southern margin of the QT (Fig. 1b). The main strata exposed in the RCA are the middle Permian Longge Formation (P2 l), Upper Triassic Riganpeicuo Formation (T3 r), Lower-Middle Jurassic Sewa Formation (J1-2 s), Lower Cretaceous MF (K1 m) and Quaternary units. P2 l is mainly composed of microcrystalline limestone and fine-grained limestone. T3 r consists mainly of micritic limestone and bioclastic limestone. J1-2 s is a complex unit of thinly bedded sandstone, medium-bedded quartzose sandstone, quartzofeldspathic sandstone and thinly bedded argillaceous slate. Notably, K1 m within the area of interest contains MF volcanic rocks, which are mainly dacite with minor andesite and rhyolite. In addition, this area contains several groups of faults and inferred faults across the basin, including NE–SW-trending, E–W-trending and nearly N–S-trending faults and/or inferred faults. The RCA contains several Mesozoic intermediate–silicic intrusions, including granodiorites, diorites and granodiorite porphyries (Fig. 1b).
The K1 m volcanic rocks are exposed mainly in the southern RCA. K1 m overlies J1-2 s and T3 r across a buried angular unconformity. Our dacite samples analysed in this study were collected from K1 m west of the Rena-Co area in the southern QT, approximately 35 km north of the BNSZ (Fig. 1b). Petrographically, although our analysed volcanic rocks in the RCA are extensively altered, the original textures appear to have been preserved. The analysed dacites in this study have a porphyritic texture, containing 20–35 vol.% phenocrysts of plagioclase (15–20 vol.%), amphibole (5–10 vol.%) and pyroxene (3–5 vol.%). The matrix is mainly oriented or interleaved plagioclase microlites, cryptocrystalline, Fe–Ti oxides and minor quartz (Fig. 2).

Figure 2. Photomicrographs showing the porphyric texture of the MF volcanic rocks collected from the RNA in the western QT, central Tibet. Note that the groundmass minerals mainly consist of plagioclase microlites, cryptocrystalline minerals and minor magnetite.
3. Results
Analytical methods, whole-rock major and trace elements, Sr-Nd-Pb isotopes and LA–ICP–MS zircon U-Pb and Lu-Hf isotope data for the RCA volcanic rocks are given in Supporting Information I. Herein, we selected the least altered samples for geochemical and isotopic analyses, with their results presented in Supporting Information II.
3.1. Major and trace elements
Whole-rock major and trace element data for the RCA volcanic rocks are presented in Table A of Supporting Information II. Based on field and petrographic observations and loss on ignition (LOI) geochemical data in the analysed samples (1.62–4.44%), with an average of 3.03 wt.%, the analysed dacite samples are relatively fresh. When normalized on a volatile-free basis, the analysed dacite samples in this study are characterized by low TiO2 contents (0.50–0.59 wt.%), low MgO contents (0.68–0.88 wt.%), low TFe2O3 contents (3.97–4.65 wt.%), low Mg# values (23.83–29.65), as well as relatively high SiO2 (64.79–70.37 wt.%), Na2O (3.79–4.70 wt.%), K2O (1.54–2.46 wt.%) and Al2O3 (13.81–16.13 wt.%) contents. The RCA dacite samples plot in the subalkaline dacite field in the SiO2 vs. Na2O + K2O diagram (Fig. 3a) and in the medium-K calc-alkaline series in the K2O vs. SiO2 diagram (Fig. 3b), with total alkalis and K2O/Na2O ratios ranging from 5.71 to 6.91 and 0.36 to 0.56, respectively. Moreover, these samples share metaluminous characteristics, with A/CNK values (molar Al2O3/[CaO+K2O+Na2O]) of 0.78–0.94 (Fig. 3c).

Figure 3. Selected plots of the MF volcanic rocks in the western QT, central Tibet. (a) Total alkalis (wt.%) vs. SiO2 (wt.%) diagram (Middlemost, Reference Middlemost1994) for classification, (b) K2O (wt.%) vs. SiO2 (wt.%) diagram (Peccerillo & Taylor, Reference Peccerillo and Taylor1976), (c) A/NK vs. A/CNK diagram (Maniar & Piccoli, Reference Maniar and Piccoli1989). Analytical data of 33 other Duolong volcanic rocks samples collected from (Wang et al. Reference Wang, Tang, Fang, Lin, Song, Wang, Yang, Yang, Li, Wei, Feng and Li2015; Sun, Reference Sun2015; Li et al. Reference Li, Qin, Li, Xiao, Zhao and Chen2016; Wei et al. Reference Wei, Tang, Song, Liu, Feng and Li2017; Shi et al. Reference Shi, Li, Huang, Liu, Huang, Li, Zhang, Ma and Li2019) are shown for debate. See text for details.
These analysed rocks show a remarkably right deviation in the chondrite-normalized rare earth element (REE) patterns (Fig. 4a), with very low total REE contents (ΣREE = 98.8–123.1 ppm) and varying enriched light rare earth elements (LaN/YbN = 18.48–20.23, where N denotes values normalized to the chondrite composition of Sun & McDonough, Reference Sun and McDonough1989). They share slightly negative to negligible Ce anomalies [δCe = 0.89–1.01, δCe = 2×CeN/(LaN+PrN)] and weakly negative Eu anomalies [δEu = 0.84–0.89, δEu = 2×EuN/(SmN+GdN)]. They exhibit high Sr (517-598 ppm) and low Y (8.45-10.7 ppm) contents, coupled with limited Sr/Y ratios (11.0–13.7). In addition, they generally show strong enrichments in large ion lithophile elements (LILEs: K, Rb, U and Pb) relative to high-field-strength elements (HFSEs: Nb, Ta, and Ti) in primitive-mantle-normalized multielement variation patterns (Fig. 4b), which are similar to the geochemical characteristics of arc-type magmas worldwide (Pearce & Peate, Reference Pearce and Peate1995; Wilson, Reference Wilson1989; Hawkesworth et al. Reference Hawkesworth, Gallagher, Hergt and Mcdermott1993).

Figure 4. Chondrite-normalized REE (a, c, e) and primitive-mantle-normalized trace element patterns (b, d, f) for the MF volcanic rocks in the western QT, central Tibet. The date sources are for the MF volcanic rocks the same as in Figure 3. N-MORB and OIB (Sun & McDonough, Reference Sun and McDonough1989). Data for normalization and plotting are from Sun & McDonough (Reference Sun and McDonough1989). Note that the MF volcanic rocks show significantly different patterns from those of N-MORB and OIB.
3.2. Zircon U-Pb ages
The zircon U-Pb dating results are listed in Table B of Supporting Information II. In cathodoluminescence (CL) images, zircons from the studied samples are mostly euhedral to subhedral in morphology. Zircons from sample RNC1610 have long axes of 50 μm to 220 μm and length-to-width ratios of 2:1 to 3:1 (Fig. 5a), while zircons from sample RNC1613 have long axes of 50 to 150 μm as well as length-to-width ratios of 1:1 to 3:1 (Fig. 5b). The CL images show that the zircon grains from these two samples have wide magmatic oscillatory zoning. The Th/U ratios of zircons range from 0.50 to 1.17 in sample RNC1610 and from 0.47 to 1.18 in sample RNC1613. Their generally high Th/U ratios, more than 0.1, indicate a magmatic origin (Corfu et al. Reference Corfu, Hanchar, Hoskin and Kinny2003; Hoskin & Schaltegger, Reference Hoskin and Schaltegger2003). Thus, zircon U-Pb ages could be interpreted as representing the eruptive age of host rocks.

Figure 5. Cathodoluminescence (CL) images of representative zircon grains and concordia plots of the MF volcanic rocks in the RCA. Solid and dashed circles indicate the locations of LA-ICP-MS U-Pb dating and Hf analyses, respectively. The scale bar length in CL image is 150μm.
After rejecting discordant ages, the 21 analyses from sample RNC1610 are generally concordant and share zircon 206Pb/238Pb ages ranging from 108.8 Ma to 110.6 Ma (Fig. 5a), with a weighted mean age of 109.5±0.6 Ma (MSWD=0.02). Similarly, the 12 analyses from sample RNC1613 are also concordant and share zircon 206Pb/238Pb ages ranging from 108.3 to 110.8 Ma (Fig. 5b), with a weighted mean age of 109.6±0.8 Ma (MSWD=0.01). Therefore, the RCA volcanic rocks were generated in the Early Cretaceous (ca. 109.5-109.6 Ma), similar to the Duolong MF volcanic rocks (105.7-118.5 Ma; Wang et al. Reference Wang, Tang, Fang, Lin, Song, Wang, Yang, Yang, Li, Wei, Feng and Li2015; Sun, Reference Sun2015; Li et al. Reference Li, Qin, Li, Xiao, Zhao and Chen2016; Wei et al. Reference Wei, Tang, Song, Liu, Feng and Li2017; Shi et al. Reference Shi, Li, Huang, Liu, Huang, Li, Zhang, Ma and Li2019) and the northern Gerze andesites (∼124 Ma; Liu et al. Reference Liu, Hu, Gao, Feng, Coulson, Feng, Qi, Yang, Yang and Tang2012).
3.3. Whole-rock Sr-Nd-Pb isotopic data
Whole-rock Sr-Nd-Pb isotopic data are listed in Table C of Supporting Information II. All samples show homogeneous Sr-Nd-Pb isotopic compositions (Fig. 6). Initial Sr, Nd and Pb isotopic ratios were corrected by the ages of 109.5 Ma and 109.6 Ma. These dacite samples have low 87Sr/86Sr(i) values (0.705119–0.705491) and exhibit a restricted range of ϵ Nd(t) values ranging from −0.28 to +1.25 (corrected to related 206Pb/238U ages), with young single-stage Nd-depleted mantle ages of TDM1 = 642 to 818 Ma. In addition, these rocks exhibit variable (206Pb/204Pb)i values of 18.489 to 18.508, (207Pb/204Pb)i values of 15.591 to 15.612 and (208Pb/204Pb)i values of 38.599 to 38.686.
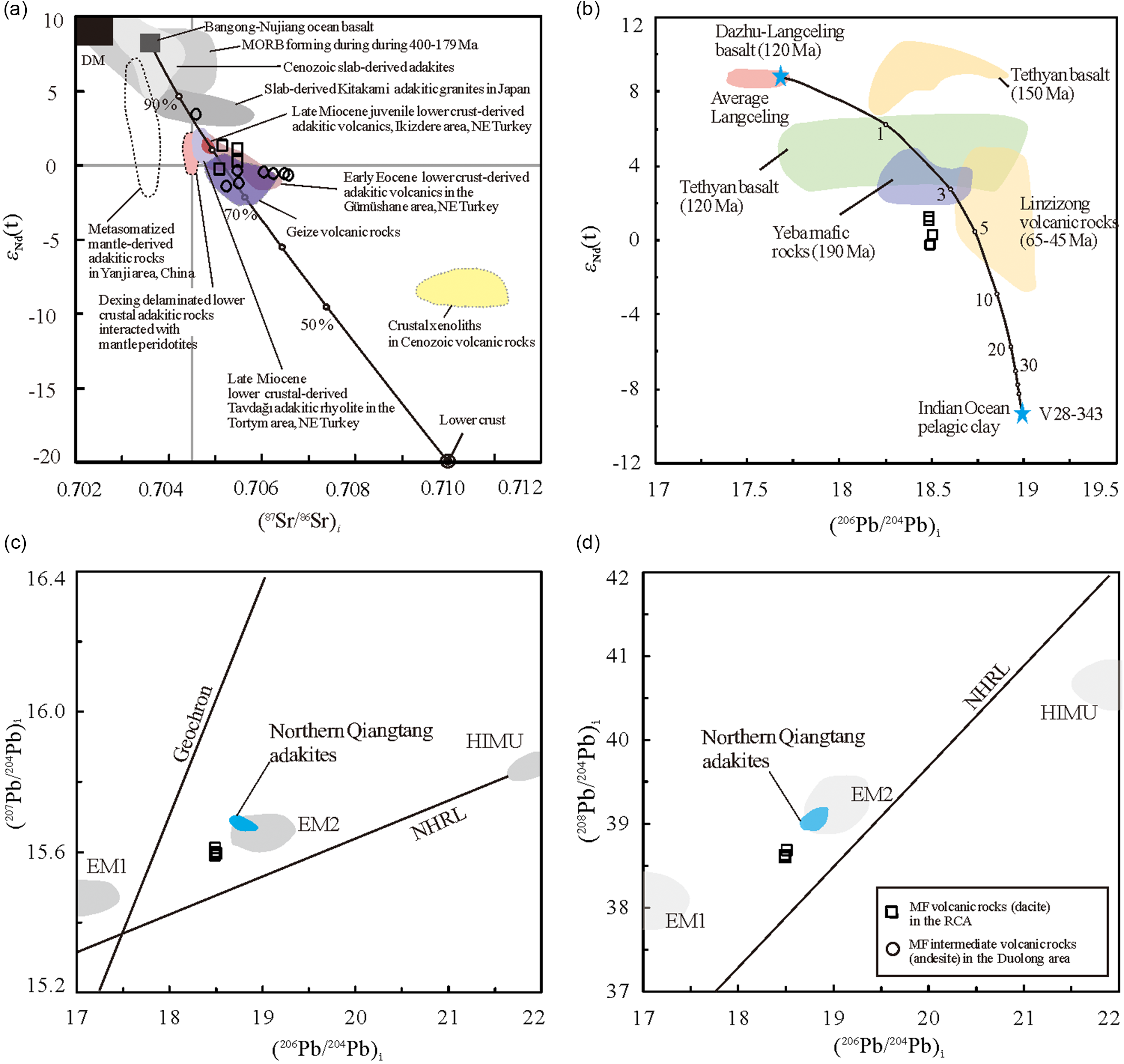
Figure 6. Whole-rock Sr, Nd and Pb isotopic compositions of the MF volcanic rocks in the western QT, central Tibet. Analytical Sr and Nd isotopic data of 12 Duolong volcanic rocks samples collected from previous studies (Sun, Reference Sun2015; Li et al. Reference Li, Qin, Li, Xiao, Zhao and Chen2016; Wei et al. Reference Wei, Tang, Song, Liu, Feng and Li2017) are shown for debate. In Panel a, data of Bangong–Nujiang Ocean basalt (Liu et al. Reference Liu, Xia, Zhong, Cai, Li, Liu, Cai and Sun2014), MORB forming during 400-179 Ma (Hofmann, Reference Hofmann and Carlson2003), the lower crust (Miller et al. Reference Miller, Schuster, Klotzli, Frank and Purtscheller1999), crustal xenoliths in Cenozoic volcanic rocks (Lai & Qin, Reference Lai and Qin2008), Dexing lower crustal derived adakitic rocks (Wang et al. Reference Wang, Xu, Jian, Bao, Zhao, Li, Xiong and Ma2006 b),Cenozoic slab-derived adakites (Defant & Kepezhinskas, Reference Defant and Kepezhinskas2001), the Gerze volcanic rocks (Liu et al. Reference Liu, Hu, Gao, Feng, Coulson, Feng, Qi, Yang, Yang and Tang2012), Slab-derived adakites in Kitakami area and Metasomatized mantle-derived adakites in Yanji area (Tsuchiya et al. Reference Tsuchiya, Suzuki, Kimura and Kagami2005), Early Eocene lower crust-derived adakitic rocks in Gümüşhane area (Karsli et al. Reference Karsli, Dokuz, Uysal, Aydin, Kandemir and Wijbrans2010), Late Miocene mafic lower crust-derived Tavdağı adakites in the Tortum area (Dokuz et al. Reference Dokuz, Uysal, Meisel, Turan, Duncan and Akçay2013) and Late Miocene juvenile lower crust-derived adakites in IKizdere area (Karsli et al. Reference Karsli, Dokuz, Kandemir, Aydin, Schmitt, Ersoy and Alyıldız2019) are shown for comparison. In Panel b, data of Tethyan basalts (150 Ma and 120 Ma; Mahoney et al. Reference Mahoney, Frei, Tejada, Mo, Leat and Nägler1998), Dazhu-Langceling basalts from the Yarlung Zangbo suture zone (Zhang et al. Reference Zhang, Mahoney, Mo, Ghazi, Milani, Crawford, Guo and Zhao2005), Indian Ocean pelagic sediment (V28-343, Ben Othman et al. Reference Ben Othman, White and Patchett1989), Yeba mafic rocks (Zhu et al. Reference Zhu, Pan, Chung, Liao, Wang and Li2008), and Linzizong andesites (Mo et al. Reference Mo, Zhao, Deng, Flower, Yu, Luo, Li, Zhou, Dong, Zhu and Wang2006, Reference Mo, Hou, Niu, Dong, Qu, Zhao and Yang2007) are shown for comparison. The star at the top in Panel b is the average value for Langceling basalts (Zhang et al. Reference Zhang, Mahoney, Mo, Ghazi, Milani, Crawford, Guo and Zhao2005), and Indian Ocean pelagic sediment is used as a proxy for oceanic sediment. In Panel c and d, NHRL is from Hart (Reference Hart1984), Geochron (4.55 Ga) and the mantle end-members HIMU, EM1 and EM2 are after Zindler & Hart (Reference Zindler and Hart1986), and Northern Qiangtang adakitic rocks are from Lai et al. (Reference Lai, Qin and Li2007). See text for details.
3.4. Zircon Hf isotopes
A total of 33 dated zircons were analysed by LA–MC–ICP–MS for Hf isotopes in this study, and the results of the initial 176Hf/177Hf(i) ratios and ϵHf(t) values are presented in Table D of Supporting Information II. These two analysed dacite samples share highly similar variations in Hf isotopes in zircons. Zircons from these dacites have uniform Hf isotopic compositions (176Hf/177Hf(i) = 0.282813 to 0.283074), yielding ϵ Hf(t) values and young Hf-depleted mantle ages (TDMC) of +3.9 to +13.1 and 331 to 923 Ma (Fig. 7), respectively.

Figure 7. Zircon ϵ Hf(t) vs. U-Pb ages diagram of the MF volcanic rocks in the western QT, central Tibet. Analytical data of 6 Duolong volcanic rocks samples collected from previous studies (Sun, Reference Sun2015; Li et al. Reference Li, Qin, Li, Xiao, Zhao and Chen2016; Wei et al. Reference Wei, Tang, Song, Liu, Feng and Li2017) are shown for debate. Inset in the diagram shows histograms of ϵ Hf(t) zircons of the MF volcanic rocks from the RCA.
4. Discussion
4.1. Tectonic setting
The dacite samples, with ages of ca. 109.5–109.6 Ma, show remarkable enrichments in light REEs (LaN/YbN = 18.48–20.23) and LILEs (e.g. Cs, Rb, U, Pb and K) but significant depletions in HFSEs (e.g. Nb, Ta and Ti), consistent with the characteristics of arc-type volcanic rocks formed in a subduction zone environment (Fig. 4a, b; Hawkesworth et al. Reference Hawkesworth, Gallagher, Hergt and Mcdermott1993; Pearce & Peate, Reference Pearce and Peate1995). In addition, they share similar geochemical signatures to the Early Cretaceous arc-type rocks related to the northern subduction of the Bangong–Nujiang oceanic lithosphere (BNOL), such as andesites from northern Gerze (ca. ∼124 Ma, Liu et al. Reference Liu, Hu, Gao, Feng, Coulson, Feng, Qi, Yang, Yang and Tang2012), Duolong MF volcanic rocks with ages of ca. 105.7–118.5 Ma (Fig. 4c, d; Wang et al. Reference Wang, Tang, Fang, Lin, Song, Wang, Yang, Yang, Li, Wei, Feng and Li2015; Sun, Reference Sun2015; Li et al. Reference Li, Qin, Li, Xiao, Zhao and Chen2016; Wei et al. Reference Wei, Tang, Song, Liu, Feng and Li2017; Shi et al. Reference Shi, Li, Huang, Liu, Huang, Li, Zhang, Ma and Li2019) and Duolong ore-bearing and barren porphyry with ages of ca. ∼116–128 Ma (e.g. She et al. Reference She, Li, Ma, Li, Zhang, Feng, Qu and Pan2009; Chen et al. Reference Chen, Zhu, Ma, Huang, Li, Li, Li, Wei and Liu2013; Li et al. Reference Li, Qin, Li, Xiao, Zhao, Cao and Chen2013 a, Reference Li, Qin, Li, Xiao, Zhao and Chen2016; Sun, Reference Sun2015; Zhu et al. Reference Zhu, Chen, Liu, Ma, Li, Zhang, Liu and Wei2015; Wei et al. Reference Wei, Song, Tang, Liu, Wang, Lin, Feng, Hou and Danzhen2018). Note that the interval between the RCA and Duolong area measures only approximately 65 kilometres spatially, forming a nearly W-S magmatic belt parallel to the BNSZ (Fig. 1). In particular, the MF volcanic rocks from the two sections yield nearly identical ages, considering their error ranges, and have essentially identical REE and trace element patterns (Fig. 4a, b; Kapp et al. Reference Kapp, Yin, Harrison and Ding2005; Chang et al. Reference Chang, Zhu, Zhao, Dong, Mo, Liu and Hu2011; Wang et al. Reference Wang, Tang, Fang, Lin, Song, Wang, Yang, Yang, Li, Wei, Feng and Li2015; Sun, Reference Sun2015; Li et al. Reference Li, Qin, Li, Xiao, Zhao and Chen2016; Wei et al. Reference Wei, Tang, Song, Liu, Feng and Li2017; Shi et al. Reference Shi, Li, Huang, Liu, Huang, Li, Zhang, Ma and Li2019; this study). Taken together, these observations show that these two sections probably share the same tectonic setting.
Interpreting the tectonic setting of altered rocks is difficult. The chemistry of some elements, particularly LILEs (e.g. K, Ba, Rb and Sr), has been easily affected by alteration, whereas elements such as Ti, V, Zr, Hf, Nb, Ta, Y, Yb and La are effectively immobile during alteration (Allègre & Minster, Reference Allègre and Minster1978; Collins et al. Reference Collins, Beams, White and Chappell1982). Therefore, the latter immobile elements should be used in interpreting the tectonic setting of the RCA dacites. When plotted on a discrimination diagram using immobile minor and trace elements, all the MF volcanic rocks from the RCA, together with those from the Duolong area, plot in the arc volcanic setting field on the Hf/3-Th-Nb/16 diagram (Fig. 8a, Wood, Reference Wood1980); this discrimination diagram could apply to both intermediate–acid rocks and basaltic rocks and is very useful for discriminating arc-related volcanic rocks (Wood et al. 1980; Hugh, Reference Hugh1993). Furthermore, the dacite samples share Ta/Yb ratios of 0.50–0.54, Nb/Yb ratios of 7.35–8.14 and Th/Yb ratios of 7.53–8.59, which plot within or near the active continental margin field rather than the within-plate field (including continental rifts and ocean islands) on the Th/Yb vs. Nb/Yb and Th/Yb vs. Ta/Yb diagrams (Fig. 8b, c; Pearce & Peate, Reference Pearce and Peate1995; Agrawal et al. Reference Agrawal, Guevara and Verma2008). Note that this is similar to the Andean arc basalts (continental island arc basalts). The Zr/Y ratios (11.02–13.70) of the RCA dacites are higher than the value of 3.0 that was used to separate the oceanic arc (Zr/Y < 3) and continental volcanic rocks (Zr/Y > 3; Pearce, Reference Pearce1982; Pearce et al. Reference Pearce, Harris and Tindle1984; Pearce & Deng, Reference Pearce and Deng1988), indicating that the RCA dacites were most likely constructed in a continental island arc setting. Moreover, they share high La/Nb ratios ranging from 3.41 to 3.79 and negative Eu anomalies ranging from 0.84 to 0.89, all of which further reinforce the subduction-related tectonic setting (Condie, Reference Condie2001). This view is also supported by the fact that these RCA volcanic rocks have high SiO2 (64.79–70.37 wt.%) and moderate K2O (1.54–2.46 wt.%) contents, with all the samples plotting in the medium-K calc-alkaline series field (Fig. 3b), given that calc-alkaline volcanic rocks have long been considered the result of subduction-related arc magmatism occurring at convergent plate boundaries (e.g. Li et al. Reference Li, He, Wang, Santosh, Dai, Zhang, Wei and Wang2013 b, Reference Li, He, Wang, Han, Ma, Xu and Du2015; and references therein).

Figure 8. Selected discrimination diagrams of the MF volcanic rocks in the western QT, central Tibet. (a) Th/Yb-Ta/Yb diagrams (Pearce, Reference Pearce1982); (b) Th/Yb-Nb/Yb diagrams (Pearce & Peate, Reference Pearce and Peate1995); (c) Hf/3-Th-Nb/16 diagram (Wood, Reference Wood1980). The date sources for the MF volcanic rocks are the same as in Figure 3. Note that the MF volcanic rocks were formed in a continental margin setting.
Hence, as presented above, the geochemical features clearly suggest that the MF dacites from the RCA possess an arc volcanic signature that corresponds with subduction, likely suggesting that they formed at a continental margin arc due to the northwards subduction of the BNOL.
4.2. Petrogenesis of the RCA dacites
As outlined above, our MF dacites from the RCA in this study contain plagioclase phenocrysts are characterized by relatively high SiO2 abundances (64.79–70.37 wt.%), high Al2O3 abundances (13.81–16.13 wt.%), high Na2O abundances (3.79–4.70 wt.%) and high Sr abundances (517-598 ppm), but low Y abundances (8.45-10.7 ppm), low Yb abundances (0.89-1.04 ppm), low Cr abundances (33.3-58.9 ppm) and low Ni abundances (17.6-33.6 ppm), with limited Sr/Y ratios of 11.0–13.7 and LaN/YbN ratios of 18.48–20.23, and they plot within the adakite field of Figure 9a. In addition, they yield weakly negative to positive whole-rock ϵ Nd(t) values (−0.28 to +1.25) and positive zircon ϵ Hf(t) values (+3.9 to +13.1). Overall, the RCA dacites display adakite-like geochemical characteristics (Fig. 9a, Defant & Drummond, Reference Defant and Drummond1990). Thus far, several hypotheses have been proposed to explain the origin of adakitic rocks, including (a) melting of subducted young and hot oceanic slabs (e.g. Stern & Kilian, Reference Stern and Kilian1996; Defant & Drummond, Reference Defant and Drummond1990; Rapp et al. Reference Rapp, Shimizu, Norman and Applegate1999; Defant et al. Reference Defant, Xu, Kepezhinskas, Wang, Zhang and Xiao2002); (b) assimilation fractional crystallization (AFC) or fractional crystallization (FC) from parental basaltic magmas (e.g. Feeley & Hacker, Reference Feeley and Hacker1995; Castillo et al. Reference Castillo, Janney and Solidum1999; Macpherson et al. Reference Macpherson, Dreher and Thirlwall2006); (c) magma mixing between felsic and basaltic magmas (e.g. Streck et al. Reference Streck, Leeman and Chesley2007); (d) melting of delaminated lower crust (e.g. Kay & Kay, Reference Kay and Kay1993; Xu et al. Reference Xu, Shinjo, Defant, Wang and Rapp2002; Gao et al. Reference Gao, Rudnick, Yuan, Liu, Liu, Xu, Lin, Ayers, Wang and Wang2004; Wang et al. Reference Wang, Wyman, Xu, Zhao, Jian, Xiong, Bao, Li and Bai2006 a, b); and (e) melting of thickened mafic lower continental crust (e.g. Atherton & Petford, Reference Atherton and Petford1993; Hou et al. Reference Hou, Gao, Qu, Rui and Mo2004; Chung et al. Reference Chung, Liu, Ji, Chu, Lee, Wen, Lo, Lee, Qian and Zhang2003, Reference Chung, Chu, Zhang, Xie, Lo, Lee, Lan, Li, Zhang and Wang2005).

Figure 9. Selected discrimination diagrams of the MF volcanic rocks in the western QT, central Tibet. (a) Sr/Y vs. Y (ppm) diagram showing data for adakites and normal calc-alkaline rocks (Defant & Drummond, Reference Defant and Drummond1990), (b) Mg# vs. SiO2 (wt.%) diagram and (c) Th/La vs. Th (ppm) diagram (Plank, Reference Plank2005). The date sources for the MF volcanic rocks are the same as in Figure 3. In Panel b, data of metabasaltic and eclogite experimental melts (1-4 Gpa) (Rapp et al. Reference Rapp, Shimizu, Norman and Applegate1999, Reference Rapp, Shimizu and Norman2003, and references therein), subducting oceanic crust-derived adakites, delaminated lower crust-derived adakites and thickened lower crust-derived adakites (Wang et al. Reference Wang, Wyman, Xu, Zhao, Jian, Xiong, Bao, Li and Bai2006 a, b, Reference Wang, Wyman, Xu, Dong, Vasconcelos, Pearson, Wan, Dong, Li, Yu, Zhu, Feng, Zhang, Zi and Chu2008 a, b, and references therein) are shown for debate. In Panel c, data of marine sediments and GLOSS (global subducting sediment) (Plank & Langmuir, Reference Plank and Langmuir1998), MORB (Niu & Batiza, Reference Niu and Batiza1997), subducted oceanic crust-derived adakites (Defant et al. Reference Defant, Xu, Kepezhinskas, Wang, Zhang and Xiao2002; Martin et al. Reference Martin, Smithies, Rapp, Moyen and Champion2005), subducting continental crust-derived adakites (Wang et al. Reference Wang, Wyman, Xu, Dong, Vasconcelos, Pearson, Wan, Dong, Li, Yu, Zhu, Feng, Zhang, Zi and Chu2008 a, b), Hohxil adakites (Wang et al. Reference Wang, McDermott, Xu, Bellon and Zhu2005, Reference Wang, Wyman, Xu, Dong, Vasconcelos, Pearson, Wan, Dong, Li, Yu, Zhu, Feng, Zhang, Zi and Chu2008 a, b) and Duogecuoren adakitic rocks (Wang et al. Reference Wang, McDermott, Xu, Bellon and Zhu2005, Reference Wang, Wyman, Xu, Dong, Vasconcelos, Pearson, Wan, Dong, Li, Yu, Zhu, Feng, Zhang, Zi and Chu2008 a, b) are shown for debate. See text for details.
We argue that the first four genetic hypotheses are not applicable to the RCA dacites based on the following observations. First, adakites from modern arcs that are interpreted as slab melts have mid-ocean-ridge basalt (MORB)-like Sr–Nd isotopic compositions and relatively low K2O, Th and Th/La values, which originate from the basaltic portion of subducting slabs (Defant & Drummond, Reference Defant and Drummond1990; Kelemen et al. Reference Kelemen, Hanghøj, Greene and Rudnick2003; Plank, Reference Plank2005). In the case of the studied adakite-like dacites from the RCA, they have more evolved Sr-Nd-Pb isotopic compositions (Fig. 6) and much higher K2O contents (1.54–2.46 wt.%), with K2O/Na2O ratios of 0.36–0.56, as well as varying higher Th values (6.9-8.6 ppm) and Th/La ratios (0.27–0.32) than those of the MORB and slab-derived (subducted oceanic crust-derived) adakites (Fig. 9c; e.g. Niu & Batiza, Reference Niu and Batiza1997; Martin et al. Reference Martin, Smithies, Rapp, Moyen and Champion2005; Wang et al. Reference Wang, Wyman, Xu, Dong, Vasconcelos, Pearson, Wan, Dong, Li, Yu, Zhu, Feng, Zhang, Zi and Chu2008 a, b; Tang et al. Reference Tang, Wang, Wyman, Li, Zhao, Jia and Jiang2010). They are geochemically distinct from the Hohxil adakites produced by melting of subducted sediments on the northwards-subducting Songpan–Ganzi oceanic slab (Fig. 9c; Wang et al. Reference Wang, McDermott, Xu, Bellon and Zhu2005, Reference Wang, Wyman, Xu, Dong, Vasconcelos, Pearson, Wan, Dong, Li, Yu, Zhu, Feng, Zhang, Zi and Chu2008 a) and the Mamen adakite-like rocks derived from partial melting of the subducted oceanic slab (MORB + sediment + fluid) (Zhu et al. Reference Zhu, Zhao, Pan, Lee, Kang, Liao, Wang, Li, Dong and Liu2009). On the other hand, if the dacites originated as subducted slab melts, they should have mid-ocean-ridge basalt-like Sr–Nd isotopic compositions (ϵ Nd(t)≈10; Defant & Drummond, Reference Defant and Drummond1990), as shown by the Bangong–Nujiang oceanic basalt (Fig. 6a; Zhu et al. Reference Zhu, Pan, Mo, Wang, Zhao and Liao2006; Liu et al. Reference Liu, Xia, Zhong, Cai, Li, Liu, Cai and Sun2014). As shown in Fig. 6a, the RCA adakite-like dacites show a more radiogenic Sr–Nd isotope signature than MORB that formed at 400-179 Ma (Hofmann, Reference Hofmann and Carlson2003), Cenozoic adakites that formed in a subducting environment (e.g. Kimura et al. Reference Kimura, Gill, Kunikiyo, Osaka, Shimoshioiri, Katakuse, Kakubuchi, Nagao, Furuyama, Kamei, Kawabata, Nakajima, van Keken and Stern2014), adakites that formed by the partial fusion of modified lithospheric mantle in the Yanji region (Guo et al. Reference Guo, Wilson and Liu2007) and adakites that originated from slab-derived melt in the Kitakami region (Tsuchiya et al. Reference Tsuchiya, Suzuki, Kimura and Kagami2005). In addition, their features of negative Nb–Ti anomalies are not consistent with N-MORB, OIB or oceanic slab sources resulting in melts, as identified by positive Nb–Ti anomalies in the spidergrams normalized to the primitive mantle (Fig. 4a, b; e.g. Sun & McDonough, Reference Sun and McDonough1989; Hofmann, Reference Hofmann1997), suggesting that their source rocks were not basaltic oceanic crust.
Second, suites of adakitic rocks derived by fractional crystallization of basaltic magmas usually exhibit variable and high Gd/Yb ratios in addition to high La/Yb and Sr/Y ratios (Macpherson et al. Reference Macpherson, Dreher and Thirlwall2006; Richards & Kerrich, Reference Richards and Kerrich2007). However, the RCA dacites exhibit low and relatively low Gd/Yb ratios (2.43–2.67), with low La/Yb ratios of 25.8 to 28.2 and Sr/Y ratios of 53.0 to 70.8. Geochemically, adakites generated by this process generally display distinct compositional trends, in which the Al2O3 and La contents decrease with increasing SiO2 contents, but the La/Y, Dy/Yb and Sr/Y ratios clearly increase with increasing SiO2 contents (Macpherson et al. Reference Macpherson, Dreher and Thirlwall2006). Nevertheless, the RCA dacites obviously exhibit none of these trends, with a relatively narrow range of SiO2 contents (64.79–70.37 wt.%). In other words, fractional crystallization can also be ruled out because of the lack of a negative linear correlation between SiO2 concentrations and Mg# values (Fig. 9b; Castillo et al. Reference Castillo, Janney and Solidum1999), as well as a lack of a positive linear correlation between SiO2 concentrations and Sr/Y and (Dy/Yb)N values (not shown; Macpherson et al. Reference Macpherson, Dreher and Thirlwall2006), for the RCA adakite-like dacites. Furthermore, these adakite-like dacites are not associated with contemporary basaltic rocks that would correspond to the required mafic end-member in AFC, FC and magma mixing models (e.g. Castillo et al. Reference Castillo, Janney and Solidum1999; Macpherson et al. Reference Macpherson, Dreher and Thirlwall2006; Streck et al. Reference Streck, Leeman and Chesley2007), indicating that they could not have been generated directly by crustal assimilation and fractional crystallization from parental basaltic magmas.
Third, it appears unlikely that they formed as a product of magma mixing between felsic and basaltic magmas, as they contain no mafic microgranular enclaves, as also evidenced by the lack of straight linear variations between the concentrations of major elements, such as MgO, K2O, Al2O3 and SiO2, within these rocks. Moreover, these samples show weakly negative to positive whole-rock ϵ Nd(t) values (Fig. 6a, b; −0.28 to +1.25) and positive zircon ϵ Hf(t) values (Fig. 7; +3.9 to +13.1), which are inconsistent with formation from magma mixing between felsic and basaltic magmas.
Fourth, their formation was not related to melting of delaminated lower crust. These RCA adakite-like dacites have whole-rock radiogenic Sr isotope [87Sr/86Sr(i) = 0.705119–0.705491], Nd isotope [ϵ Nd(t) of −0.28 to +1.25] and Pb isotope [(206Pb/204Pb)i = 18.489–18.508, (207Pb/204Pb)i = 15.591–15.612, (208Pb/204Pb)i = 38.599–38.686] compositions (Fig. 6b, c, d), which are remarkably different from the crustal xenoliths in Cenozoic volcanic rocks from the QT, northern Tibet (Fig. 6a; Lai & Qin, Reference Lai and Qin2008). As mentioned above, their geochemical and Sr–Nd isotopic features significantly differ from those of the Cenozoic crust-derived adakites of the Songpan–Ganzi block (Fig. 6c, d; Wang et al. Reference Wang, Wyman, Xu, Dong, Vasconcelos, Pearson, Wan, Dong, Li, Yu, Zhu, Feng, Zhang, Zi and Chu2008 a), the Mesozoic Anjishan adakites from the Ningzhen area of East China generated by partial melting of delaminated lower continental crust [ϵ Nd(t) = −6.8 to −9.7, (87Sr/86Sr)i = 0.7053 to 0.7066, Xu et al. Reference Xu, Shinjo, Defant, Wang and Rapp2002], the Zougouyouchaco and Dogai Coring adakitic volcanic rocks in the southern and middle Qiangtang region that were most likely derived from partial melting of delaminated lower continental crust [ϵ Nd(t) = −3.8 to −5.0, (87Sr/86Sr)i = 0.706–0.708; Liu et al. Reference Liu, Hu, Feng, Zhou, Li, Chi, Peng, Zhong, Qi, Qi and Wang2008], and the Dexing adakites originating from the partial fusion of delaminated lower crust that experienced a melt and mantle peridotite interaction (Wang et al. Reference Wang, Xu, Jian, Bao, Zhao, Li, Xiong and Ma2006 b). Moreover, their low Mg# values (23.83–29.65) and low MgO abundances (0.68–0.88 wt.%) also argue against the origin of melting of delaminated lower crust (Fig. 9b).
We suggest that the RCA adakite-like dacite suites were probably generated by melting of juvenile mafic lower continental crust. Notably, their typical geochemical magmatic arc signatures, such as enrichment in LILEs, depletion in HFSEs, especially negative Nb–Ta–Ti anomalies, and light REE (LREE)-enriched chondrite-normalized REE patterns (LaN/YbN = 18.48–20.23), with relatively negative Eu anomalies (δEu = 0.84–0.89), are consistent with those of rhyolites in the Rena-Co area (ca. 110 Ma), which are proposed to be derived from juvenile crust (Chang et al. Reference Chang, Zhu, Zhao, Dong, Mo, Liu and Hu2011). In particular, they show relatively low MgO and Mg# values and Cr and Ni contents, which are very comparable with those of adakitic rocks originating from the anatexis of the thickened lower crust (Castillo, Reference Castillo2012). Melting experiments of natural hydrous basalts under 0.8–3.2 GPa have revealed that pristine melts of basaltic rocks typically display low MgO and Mg# values (generally lower than 43; Rapp & Watson, Reference Rapp and Watson1995). As mentioned earlier, the RCA adakite-like dacites yield low MgO and Mg# values, similar to those of the pure experimental melts, which further confirms an origin of juvenile lower crustal melts for their generation (Fig. 9b; e.g. Rapp et al. Reference Rapp, Shimizu, Norman and Applegate1999, Reference Rapp, Shimizu and Norman2003; Rapp & Watson, Reference Rapp and Watson1995).
They display low initial 87Sr/86Sr(i) values of 0.705119 to 0.705491, weak negative to positive whole-rock ϵ Nd(t) values of −0.28 to +1.25 and positive zircon ϵ Hf(t) values from +3.9 to +13.1, which are features that might have been inherited from juvenile crustal magmas. As shown in Figure 6a, the adakitic dacites have Sr–Nd isotope signatures identical to those of the late Miocene juvenile lower crust-derived adakitic volcanics in the Ikizdere area (Karsli et al. Reference Karsli, Dokuz, Kandemir, Aydin, Schmitt, Ersoy and Alyıldız2019), the late Miocene mafic lower crust-derived Tavdağı adakitic rhyolite in the Tortum area (Dokuz et al. Reference Dokuz, Uysal, Meisel, Turan, Duncan and Akçay2013) and the early Eocene lower crust-derived adakitic volcanics in the Gümüşhane area (Karsli et al. Reference Karsli, Dokuz, Uysal, Aydin, Kandemir and Wijbrans2010). Furthermore, the adakite-like dacites share relatively young T DM2 ages ranging from 806 to 931 Ma and Palaeozoic and Neoproterozoic Hf crustal model ages of 332 to 924 Ma, possibly suggesting that they were likely inherited from the melts of juvenile basaltic crust, which in turn was derived from old depleted mantle (van de Zedde & Wortel, Reference van de Zedde and Wortel2001). This view is also supported by the fact that these samples have much more enriched whole-rock Sr–Nd isotopic compositions and more depleted zircon Hf isotopic compositions than those of the existing Jurassic intrusive rocks in the southern QT, such as the Larelaxin, Caima, Qingcaoshan, Charkang-Co and Rena-Co plutons, which are considered to be generated by partial melting of ancient lower continental crustal materials or by the mixing of crust-derived and mantle-derived melts (e.g. Li et al. Reference Li, Qin, Li, Richards, Zhao and Cao2014 a, b; Hao et al. Reference Hao, Wang, Wyman, Ou, Dan, Jiang, Wu, Yang, Long and Li2016 a, b; and references therein).
Note that zircons from the RCA adakite-like dacite samples show large variations in their ϵ Hf(t) values (up to 7.8 and 9.2 ϵ units for the two samples, respectively), thereby indicating an open magmatic system process (Griffin et al. Reference Griffin, Wang, Jackson, Pearson, O’Reilly, Xu and Zhou2002; Kemp et al. Reference Kemp, Hawkesworth, Foster, Paterson, Woodhead, Hergt, Gray and Whitehouse2007; Chiu et al. Reference Chiu, Chung, Wu, Liu, Liang, Lin, Iizuka, Xie, Wang and Chu2009). This hypothesis is also supported by highly radiogenic Pb isotopic compositions between mantle and crust xenoliths (Fig. 6b, c, d; Lai & Qin, Reference Lai and Qin2008), likely indicating a mixing trend. The (207Pb/204Pb)i values of 15.591–15.612 and (208Pb/204Pb)i values of 38.599–38.686 in the rocks are unusually radiogenic and plot well above the Northern Hemisphere Reference Line (NHRL) (Hart, Reference Hart1984), shifting to higher (206Pb/204Pb)i values of 18.489–18.508 than the Geochron (4.55 Ga) (Fig. 6c, d). In addition, their ϵ Hf(t) values (+3.9 to +13.1; n = 33) are similar to those of the Duolong Early Cretaceous volcanic rocks and intrusive rocks that are considered to be derived from juvenile lower crust (e.g. She et al. Reference She, Li, Ma, Li, Zhang, Feng, Qu and Pan2009; Xin et al. Reference Xin, Qu, Wang, Liu, Zhao and Huang2009; Chen et al. Reference Chen, Zhu, Ma, Huang, Li, Li, Li, Wei and Liu2013; Li et al. Reference Li, Qin, Li, Xiao, Zhao, Cao and Chen2013 a, Reference Li, Qin, Li, Xiao, Zhao and Chen2016; Sun Reference Sun2015; Zhu et al. Reference Zhu, Chen, Liu, Ma, Li, Zhang, Liu and Wei2015; Wei et al. Reference Wei, Tang, Song, Liu, Feng and Li2017, Reference Wei, Song, Tang, Liu, Wang, Lin, Feng, Hou and Danzhen2018). Notably, these volcanic rocks from the RCA show lower zircon ϵ Hf(t) values than depleted mantle (Fig. 7), possibly implying at least partial contributions from metasomatized peridotite with partial melts of subduction-related fluids into their magma source (Woodhead et al. Reference Woodhead, Hergt, Davidson and Eggins2001; Barry et al. Reference Barry, Pearce, Leat, Millar and Le Roex2006). Such a foregoing interpretation is consistent with the modelling curves defined by the MF adakite-like rocks from the RCA in Sr–Nd and Nd–Pb isotope diagrams (Fig. 6a, b). Consequently, we consider that the RCA volcanic rocks contain a great proportion of approximately 75–80% mantle-derived material contributions, according to their Sr–Nd isotopic compositions (Fig. 6a), as well as melts of ∼3–5% subduction-related fluids, according to their Nd–Pb isotopic compositions (Fig. 6b), given that a small contribution of fluids can sensitively cause a drastic increase in 206Pb/204Pb ratios in subduction-related rocks (Vroon et al. Reference Vroon, van Bergen, Klaver and White1995; Rolland et al. Reference Rolland, Picard, Pecher, Lapierre, Bosch and Keller2002). In particular, the same observations occurred for igneous rocks of the Cretaceous Ladakh arc (Rolland et al. Reference Rolland, Picard, Pecher, Lapierre, Bosch and Keller2002) and the Cretaceous Kohistan island arc (Bignold & Treloar, Reference Bignold and Treloar2003), of which several percent of subduction-related fluids became entrained into the magma source regions, in accordance with the measured Sr-Nd-Pb isotopic compositions.
In summary, these observations lead us to infer that the RCA adakite-like dacites were derived from melting of juvenile thickened mafic lower continental crust that contains melts of metasomatized peridotite and subduction-related fluids in the magma source region, likely as a result of the northern subduction of the BNOL beneath the southern QT.
4.3. Geodynamic implications
As one of the ancient orogens in the Tibetan Plateau, the BNSZ extending along central Tibet represents the oceanic remnant of the Bangong–Nujiang Tethyan Ocean (e.g. Allègre et al. Reference Allègre, Courtillot, Tapponnier, Hirn, Mattauer, Coulon, Jaeger, Achache, Schärer, Marcoux, Burg, Girardeau, Armijo, Gariépy, Göpel, Li, Xiao, Chang, Li, Lin, Teng, Wang, Chen, Han, Wang, Den, Sheng, Cao, Zhou, Qiu, Bao, Wang, Wang, Zhou and Xu1984; Hsu et al. Reference Hsu, Pan and Sengor1995; Pearce & Deng, Reference Pearce and Deng1988; Yin & Harrison, Reference Yin and Harrison2000; Kapp et al. Reference Kapp, Yin, Manning, Harrison and Taylor2003, Reference Kapp, Yin, Harrison and Ding2005, Reference Kapp, DeCelles, Gehrels, Heizler and Ding2007). Zhu et al. (Reference Zhu, Li, Cawood, Wang, Zhao, Liu and Wang2016) argued a model in which the Bangong Ocean may have closed through arc–arc ‘soft’ collision driven by divergent double-sided subduction rather than continent–continent ‘hard’ collision. Some studies have argued that the BNO closed between the Late Jurassic and the Early Cretaceous (Yin & Harrison Reference Yin and Harrison2000; Kapp et al. Reference Kapp, Yin, Harrison and Ding2005; Sui et al. Reference Sui, Wang, Zhu, Zhao, Chen, Santosh, Hu, Yuan and Mo2013; Wang et al. Reference Wang, Wang, Chung, Chen, Yin, Liu, Li and Chen2016; Zhu et al. Reference Zhu, Li, Cawood, Wang, Zhao, Liu and Wang2016; Hu et al. Reference Hu, Ma, Xue, Garzanti, Cao, Li, Sun and Lai2022). However, numerous recent studies that have focused on radiolarians, ophiolitic fragments and mélanges within the BNSZ (Zhu et al. Reference Zhu, Pan, Mo, Wang, Zhao and Liao2006; Bao et al. Reference Bao, Xiao, Su and Wang2007; Baxter et al. Reference Baxter, Aitchison and Zyabrev2009; Fan et al. Reference Fan, Li, Xie and Wang2014; Zhang et al. Reference Zhang, Xia, Zhang, Liu, Zeng, Li and Xu2014) suggest that the BNO was still open during the Early Cretaceous and that the final Lhasa–Qiangtang amalgamation occurred in the Late Cretaceous (approximately 100 Ma; e.g. Baxter et al. Reference Baxter, Aitchison and Zyabrev2009; Fan et al. Reference Fan, Li, Xie and Wang2014) rather than in the Late Jurassic–Early Cretaceous (e.g. Xu et al. Reference Xu, Schärer and Allègre1985; Dewey et al. Reference Dewey, Shackleton, Chang and Sun1988; Yin et al. Reference Yin, Xu, Liu and Li1988; Kapp et al. Reference Kapp, Yin, Manning, Harrison and Taylor2003, Reference Kapp, Yin, Harrison and Ding2005, Reference Kapp, DeCelles, Gehrels, Heizler and Ding2007; Raterman et al. Reference Raterman, Robinson and Cowgill2014), as also exemplified by the abundant Early Cretaceous magmatic rocks in the southern QT, which are interpreted as having formed in a continental arc setting (e.g. Kapp et al. Reference Kapp, Yin, Harrison and Ding2005, Reference Kapp, DeCelles, Gehrels, Heizler and Ding2007; Chang et al. Reference Chang, Zhu, Zhao, Dong, Mo, Liu and Hu2011; Liu et al. Reference Liu, Hu, Gao, Feng, Coulson, Feng, Qi, Yang, Yang and Tang2012; Hao et al. Reference Hao, Wang, Wyman, Ou, Dan, Jiang, Wu, Yang, Long and Li2016 a, b; Wang et al. Reference Wang, Tang, Fang, Lin, Song, Wang, Yang, Yang, Li, Wei, Feng and Li2015; Sun, Reference Sun2015; Li et al. Reference Li, Qin, Li, Xiao, Zhao, Cao and Chen2013 a, b, Reference Li, He, Wang, Han, Ma, Xu and Du2015, Reference Li, Qin, Li, Xiao, Zhao and Chen2016; Wei et al. Reference Wei, Tang, Song, Liu, Feng and Li2017, Reference Wei, Song, Tang, Liu, Wang, Lin, Feng, Hou and Danzhen2018; Shi et al. Reference Shi, Li, Huang, Liu, Huang, Li, Zhang, Ma and Li2019; this study). Stratigraphic analysis suggests that the QT underwent N–S-directed compression and significant crustal shortening during the Early Cretaceous (Murphy et al. Reference Murphy, Yin, Harrison, Dürr, Chen, Ryerson, Kidd, Wang and Zhou1997; Kapp et al. Reference Kapp, Yin, Harrison and Ding2005, Reference Kapp, DeCelles, Gehrels, Heizler and Ding2007), probably as a result of the collision of an oceanic plateau with the Qiangtang continental margin (Zeng et al. Reference Zeng, Xu, Chen, Wang, Huang, Xia and Li2021). It has long been recognized that the BNOL subducted northwards beneath the southern QT before the closure of the BNO (e.g. Allègre et al. Reference Allègre, Courtillot, Tapponnier, Hirn, Mattauer, Coulon, Jaeger, Achache, Schärer, Marcoux, Burg, Girardeau, Armijo, Gariépy, Göpel, Li, Xiao, Chang, Li, Lin, Teng, Wang, Chen, Han, Wang, Den, Sheng, Cao, Zhou, Qiu, Bao, Wang, Wang, Zhou and Xu1984; Yin & Harrison, Reference Yin and Harrison2000; Guynn et al. Reference Guynn, Kapp, Pellen, Heizer, Gehrels and Ding2006; Kapp et al. Reference Kapp, Yin, Manning, Harrison and Taylor2003, Reference Kapp, Yin, Harrison and Ding2005, Reference Kapp, DeCelles, Gehrels, Heizler and Ding2007; Zhu et al. Reference Zhu, Li, Cawood, Wang, Zhao, Liu and Wang2016; Wei et al. Reference Wei, Song, Tang, Liu, Wang, Lin, Feng, Hou and Danzhen2018). Our data reveal the presence of adakite-like dacites with ages of 109.5–109.6 Ma in the RCA, approximately 30 km to the north of the BNSZ (Fig. 1b). These adakite-like dacites formed from magmas generated by melting of juvenile lower crustal material and have compositions consistent with adakites that formed in a continental marginal arc. Together with previous data on the Early Cretaceous intrusive and volcanic rocks (especially the Duolong Meriqiecuo volcanic rocks) distributed in the southern QT (She et al. Reference She, Li, Ma, Li, Zhang, Feng, Qu and Pan2009; Xin et al. 2009; Liu et al. Reference Liu, Hu, Gao, Feng, Coulson, Feng, Qi, Yang, Yang and Tang2012; Chen et al. Reference Chen, Zhu, Ma, Huang, Li, Li, Li, Wei and Liu2013; Li et al. Reference Li, Qin, Li, Xiao, Zhao, Cao and Chen2013 a, Reference Li, Qin, Li, Xiao, Zhao and Chen2016; Wang et al. Reference Wang, Tang, Fang, Lin, Song, Wang, Yang, Yang, Li, Wei, Feng and Li2015; Sun, Reference Sun2015; Zhu et al. Reference Zhu, Li, Cawood, Wang, Zhao, Liu and Wang2016; Wei et al. Reference Wei, Tang, Song, Liu, Feng and Li2017, Reference Wei, Song, Tang, Liu, Wang, Lin, Feng, Hou and Danzhen2018; Shi et al. Reference Shi, Li, Huang, Liu, Huang, Li, Zhang, Ma and Li2019), we confidently propose that the sources of the RCA adakite-like magmas were most likely associated with crustal thickening, given that crustal thickening is a common geological phenomenon in arc settings. All of this evidence indicates that the timing of the final closure of the BNO might have been later than the Early Cretaceous (109.5.9-109.6 Ma).
Recently, Hao et al. (Reference Hao, Wang, Wyman, Ou, Dan, Jiang, Wu, Yang, Long and Li2016 a, b) also suggested that ancient lower crust was gradually replaced by younger materials between the Late Jurassic and Early Cretaceous and finally resulted in vertical crustal growth by magma underplating for the southern Qiangtang continental arc, based on the tracking of the magma sources of the late Mesozoic intermediate–felsic intrusive rocks in southern Qiangtang. Generally, such magma underplating is considered a result of asthenospheric upwelling, which is related to oceanic subduction with a slab rollback component due to drag and gravity forces (Nakakuki & Mura, Reference Nakakuki and Mura2013). As discussed above, the magma of the RCA adakite-like dacites most likely originated from melts of juvenile thickened lower crust. In this case, we suggest that a slab rollback model explains the magmatic flare-up that occurred in the southern QT during the Early Cretaceous and accounts for the formation of the RCA adakite-like dacites (ca. 109.5-109.6 Ma). This mechanism may also apply to the geological processes in the northern Lhasa and Tethyan Himalayan thrust belts (Raterman et al. Reference Raterman, Robinson and Cowgill2014; Zhu et al. Reference Zhu, Li, Cawood, Wang, Zhao, Liu and Wang2016), both of which represent passive margins on the lower subducting plate of a collision zone.
In this model, the downgoing BNOL carried subducted fluids into the deep mantle during its northwards subduction and then resulted in the initiation of partial melting of mantle peridotite (Elliott et al. Reference Elliott, Plank, Zindler, White and Bourdon1997). Subsequently, variable degrees of partial melts of mantle peridotite metasomatized by subducted fluids moved upwards, and basaltic underplating gradually replaced the ancient lower crust and accumulated to form juvenile basaltic crust. Thus, as a result of Early Cretaceous slab rollback, this newly underplated juvenile basaltic lower crust was further heated by a flow of upwelling hot asthenospheric materials (van de Zedde & Wortel, Reference van de Zedde and Wortel2001; Zhu et al. Reference Zhu, Li, Cawood, Wang, Zhao, Liu and Wang2016; Li et al. Reference Li, He, Wang, Santosh, Dai, Zhang, Wei and Wang2013 b, Reference Li, He, Wang, Han, Ma, Xu and Du2015), finally leading to the generation of primary basaltic magmas for the RCA adakite-like dacites (Fig. 10; Richards, Reference Richards2003; Zhu et al. Reference Zhu, Pan, Chung, Liao, Wang and Li2008).

Figure 10. Schematic illustrations showing the geodynamic evolution of the Bangong–Nujiang oceanic northern subduction beneath QT during the Early Cretaceous (modified from Richards (Reference Richards2003) and Zhu et al. (Reference Zhu, Pan, Chung, Liao, Wang and Li2008)). Note that a slab rollback model combining Bangong–Nujiang oceanic subduction and underplating of mafic magmas proposed for the generation of the Early Cretaceous Meiriqiecuo magmatism in the southern QT. See text for details.
5. Conclusions
-
(1) Zircon U-Pb dating suggests that the RCA dacites formed at 109.5.9-109.6 Ma, marking Late Cretaceous volcanic magmatism during the northern subduction of the BNOL beneath the southern QT.
-
(2) They exhibit geochemical features with adakite affinity and most likely originated from melts of the juvenile continental lower crust, which contains melts of metasomatized peridotite and subduction-related fluids in the magma source region.
-
(3) Based on previous studies and our new data, we propose that a slab rollback model could explain the formation of the RCA adakite-like dacites, which was most likely associated with crustal thickening.
Supplementary material
To view supplementary material for this article, please visit https://doi.org/10.1017/S0016756823000274
Acknowledgements
This research was supported by Research grants from National Institute of Natural Hazards, Ministry of Emergency Management of China (Grant Number: ZDJ2019-01) and the Program of the China Geological Survey (Grant Number: DD20190167). We thank the laboratorians for helping with zircon U-Pb age and Lu-Hf isotopic, whole-rock geochemical and Sr-Nd-Pb isotopic analysis. Besides, we also thank two anonymous reviewers for their constructive reviews that improved the quality of this manuscript.
Declaration of Competing Interest
The authors declare that they have no known competing financial interests or personal relationships that could have appeared to influence the work reported in this paper.