Higher intake of fruit and vegetables (F&V) reduces the risk of chronic diseases including CVD(Reference Crowe, Roddam and Key1, Reference Hung, Joshipura and Jiang2), diabetes(Reference Bazzano, Serdula and Liu3), stroke(Reference He, Nowson and MacGregor4, Reference Joshipura, Ascherio and Manson5) and cancer(6). It is also linked to a lower risk of obesity(Reference Rolls, Ello-Martin and Tohill7) and hypertension(Reference Appel, Moore and Obarzanek8). Despite this, low F&V intake is common in many countries(Reference Hall, Moore and Harper9). Among high-income countries, low F&V consumption is more prevalent in those of lower socio-economic status(Reference Giskes, Turrell and Patterson10–Reference Dehghan, Akhtar-Danesh and Merchant12). Successful strategies to increase F&V intake offer the potential to reduce the economic costs and prevalence of chronic diseases. Strategies that are effective in those of low socio-economic status have the potential to also reduce health inequalities.
Extensive health promotion campaigns to promote F&V consumption have not been sufficient to reduce the high prevalence of less healthy dietary patterns(Reference Lock, Pomerleau and Causer13). Pricing strategies, including both taxation of less healthy foods and price discounts or subsidies for healthier foods, have been proposed as alternative strategies that could complement health promotion(Reference Cobiac, Vos and Veerman14). Economic modelling consistently indicates that price subsidies for healthy foods are likely to produce significant improvements in healthy food consumption, including F&V consumption(Reference Sassi, Cecchini and Lauer15, Reference Jensen and Smed16), particularly in those on low incomes(Reference Smed, Jensen and Denver17, Reference Powell and Chaloupka18). In the USA and the UK, where long-standing food subsidy programmes are important components of social security for low-income families, there have been recent changes made to some of these programmes to better promote the consumption of F&V. Despite the large investment in food subsidy programmes, particularly in the USA, there is limited high-quality evidence of their effectiveness(Reference D'Souza, Renfrew and McCormick19). A 12·5 % price discount, but not tailored nutrition education, increased healthy food purchases by 11 % in one randomised controlled trial (RCT) in a supermarket setting(Reference Ni Mhurchu, Blakely and Jiang20). Another RCT, currently underway, will enhance these findings by comparing skill building for healthy eating with or without price discounts(Reference Ball, McNaughton and Mhurchu21).
In Australia, Aboriginal and Torres Strait Islander people have poorer health outcomes and are more likely to be of lower socio-economic status than the general population(22). Pricing strategies to promote healthy nutrition have not been widely implemented in Australia, either among Indigenous Australians or among those with low incomes. In this context, the present study reports on a F&V subsidy programme instituted at three rural Aboriginal community-controlled health services. The programme was initially instituted in 2005 at one service in response to poor nutrition among low-income families visiting the health service. The aims of the programme were to improve nutritional intake and engage participants in preventive health activities.
The aim of the present study was to evaluate whether participation in this F&V subsidy programme for 12 months was associated with changes in nutritional biomarker levels and dietary behaviour in a cohort of Aboriginal children.
Subjects and methods
The fruit and vegetable subsidy programme
A F&V subsidy programme was established by the Bulgarr Ngaru Medical Aboriginal Corporation for the Aboriginal communities in rural towns in the Clarence Valley in New South Wales, Australia, in 2005. The health service invited low-income Aboriginal families with one or more young children to join the programme, which combined annual health assessments, including dental and hearing check-ups, with weekly receipt of a box of subsidised F&V. The boxes of seasonal F&V were organised by local F&V shops in each community at a family's request. Each family paid five dollars for a box containing $40 worth of F&V (or $60 if five or more children in the household) and collected the box from the F&V shop. The F&V boxes contained a selection of commonly available F&V (with more vegetables than fruit) chosen by the F&V shops based on value for money and availability (see Supplementary file 1 (available online) for a list of options provided to guide the F&V shop staff). Families could exchange some unwanted F&V for alternative F&V. The programme was developed after the success of a nutrition programme in a remote Aboriginal community school in the Clarence Valley, described elsewhere(Reference Jones and Smith23, Reference Jones and Smith24).
This is an ongoing programme in the Clarence Valley; however, this evaluation study involved all new families who joined the programme at this time. At baseline (before receiving F&V) and after 12 months, all children in these families underwent assessments of dietary intake and nutritional status specifically as part of the evaluation. The recruitment and baseline assessments were undertaken between December 2008 and September 2009, with follow-up assessments being completed between December 2009 and September 2010.
Additional funding enabled the Galambila Aboriginal Health Service in Coffs Harbour and the Giingan Darrunday Marlaanggu Health Clinic in the Nambucca Valley to institute similar F&V subsidy programmes for the duration of this evaluation study. These health services decided to participate in this evaluation study, and all families at these locations participated in the nutritional status assessments for this evaluation. Although the demographics and health status are similar in each of these Aboriginal communities, the availability of and arrangements with the F&V shops varied in the communities. In Coffs Harbour, families received vouchers from the health service, which they redeemed at the F&V shop by selecting their own F&V. In the Nambucca Valley, the F&V shop was in a different town, hence the health service staff collected and delivered the boxes of F&V to families at their homes and collected the $5 contribution from them.
Nutrition promotion complemented the F&V boxes with seasonal recipe and practical cooking and nutrition education sessions conducted by dietitians (in the Clarence Valley and the Nambucca Valley) or trained nutrition health workers (in Coffs Harbour). There were three or four of these sessions conducted over 12 months in each of the towns in the Clarence Valley, although attending the sessions was not mandatory and less than half of the participating families attended the sessions. There were five sessions conducted over 5 weeks in the Nambucca Valley, which were well attended. No group education occurred in Coffs Harbour; however, individual families were given nutrition education after the children's 24 h dietary recalls by the nutrition health workers. Simple seasonal recipes were shared between sites, although the content of nutrition education was determined by the local staff.
Participants
The participants were low-income Aboriginal families (predominantly unemployed or receiving pensions) with one or more children aged ≤ 17 years (at commencement). Many of the children had identified nutrition risk (e.g. underweight or overweight and chronic or recurrent infections) or presented frequently with episodes of illness to the health service. Parents/carers provided consent and agreed for their children to undergo annual health assessments including the research evaluation assessments.
Data collection and laboratory methods
As part of the annual health assessments, each child's nutritional status was assessed with a 24 h dietary recall and a blood test before and after 12 months on the programme. These assessments were completed as close to 1 year apart as possible to minimise seasonal variation. Blood samples were analysed for carotenoid, vitamin A, vitamin E, vitamin C, lipid and C-reactive protein levels. Individual results were discussed with the participant/carer at follow-up appointments, and overall summary results were presented in a non-technical language to the communities during focus groups.
The 24 h dietary recalls were completed by the participants face to face with a dietitian or a trained Aboriginal health worker. For participants aged < 10 years, these were completed together with a parent/carer. Data collection was undertaken by following the multi-pass method described in the 1995 Australian National Nutrition Survey(Reference McLennan and Podger25). In addition, the participants were asked about their usual consumption of F&V using validated short dietary questions based on the Dietary Intake Assessment Tool developed in Queensland, Australia(Reference Flood, Webb and Rangan26). Short dietary questions have been developed for use in children's dietary surveys(Reference Flood, Webb and Rangan26) and were used in the present study to contrast with the estimates of self-reported F&V intake from the 24 h recalls.
Non-fasting venous blood samples were collected by clinical staff into tubes wrapped in a foil and stored on ice before transport to a local pathology laboratory. In the laboratory, they were separated, and plasma samples for the measurement of carotenoid, vitamin A and vitamin E levels were frozen at − 20°C. The frozen plasma samples were transported on dry ice and stored at − 80°C before analysis. The samples were protected from light throughout processing to prevent carotenoid degradation.
Blood samples were analysed for the levels of lipids and C-reactive protein at either Grafton Hospital Pathology, on a Cobas Integra 800 chemistry analyser (Roche Diagnostics), or Symbion Laverty Pathology, Coffs Harbour, on a Cobas Integra 400 chemistry analyser (Roche Diagnostics). Frozen plasma samples were transported on dry ice to Royal Prince Alfred Hospital, Sydney, and were analysed for vitamin C level using HPLC with electrochemical detection. Due to logistical problems, samples for the measurement of vitamin C level were not obtained at baseline in Coffs Harbour or the Nambucca Valley.
The levels of carotenoids, retinol and tocopherols were analysed using HPLC, as described by Su et al. (Reference Su, Rowley and O'Dea27) with minor changes. Briefly, after extraction and drying, 50 μl of a plasma mixture were analysed using HPLC (Shimadzu HPLC machine equipped with an SPD-M20A PDA detector and a NovoPak C18 column; Shimadzu Corporation) with the detection of absorbance at 292 nm for tocopherols, 325 nm for retinol and 450 nm for carotenoids. The samples were assayed using standardised techniques by laboratory staff who were unaware of the study design.
The dietary recall data were analysed using the FoodWorks Professional 2009 dietary software programme (Xyris) using the AusNut 2007 Australian food database. Data entry and analysis were completed by a post-graduate dietetics student with input from an experienced dietetics researcher.
Statistical analysis
The analysis sought to answer the question of whether the F&V programme was associated with changes in biomarker levels and dietary behaviour, and the primary outcomes analysed were the mean change in the levels of each carotenoid. The changes between the follow-up data and the baseline data for each participant were compared using paired t tests and the general linear model function in SPSS version 19 (IBM). The analysis was based on completed observations. Outcomes were assessed by comparing the change between the pre-levels and the post-levels, which increased the likelihood of these outcomes being normally distributed and checked for normality before undertaking parametric analyses. The crude changes in biomarker levels and dietary outcomes are presented as means and 95 % CI using paired t tests. These outcomes were adjusted by age, sex and community using a general linear model to account for differences due to age, sex or community. A 95 % CI of the mean of the difference for each outcome that did not cross zero indicated a statistically significant difference. The effect of additional covariates in the statistical model was assessed, including total cholesterol and baseline values for biomarkers and baseline values for dietary outcomes. Based on previous studies, the sample size required to detect the expected mean changes of 10–50 % in the levels of these carotenoids with a statistical significance level of 5 % and power of 80 % ranged from 9 for β-cryptoxanthin to 108 for lycopene(Reference Rose, Habicht and Devaney28, Reference Rowley, Su and Cincotta29). Estimated sample sizes accounted for the family-level design effect, assuming an average family size of 2·6 with a family-level intra-class correlation coefficient of 0·5(Reference Metcalf, Scragg and Stewart30). Given the exploratory nature and hypothesis-generating intentions of the study, analyses were not adjusted for the design effect or for multiple comparisons. Not accounting for multiple comparisons was deemed to be justified on the basis of our intention to estimate the extent of changes in biomarker levels, consequent to the introduction of a fruit/vegetable intervention, rather than to formally test specific hypotheses as to whether changes occurred. Our statistical procedures reflect this focus on estimation rather than on hypothesis testing.
Ethics
The present study was conducted according to the guidelines laid down in the Declaration of Helsinki, and all procedures involving human subjects were approved by the human research ethics committees of the University of Melbourne, the University of South Australia and the Aboriginal Health and Medical Research Council (New South Wales). Community consent was obtained from the Boards of the three participating health services. The North Coast Area Health Service Research Ethics Committee approved the clinical audits of the participants' hospital records. Written informed consent was obtained from all subjects/parents or guardians.
Results
There were fifty-five Aboriginal families, which included 174 children, recruited to participate in the evaluation of the F&V programme at the three Aboriginal Health Services. All assessments were of these children aged 0–17 years at baseline, with the majority of the children being aged between 3 and 12 years. The demographic characteristics of the participants at baseline are given in Table 1. As shown in Table 1, this was a cohort of low-income families with the majority receiving government benefits as their main source of income. The majority of adults in these households were smokers. There were differences between the cohorts of families at each location: the children were older and a small proportion of adults smoked in Coffs Harbour, while the children were younger and a greater proportion were female in the Nambucca Valley. However, the sample size at each location was not sufficient to examine the impact of these differences or differences in the implementation of the programme between the communities.
Table 1 Baseline demographic characteristics of the participating children by individual community (n 174) (Mean values and standard deviations; number of participants and percentages)

* Percentage of those with a valid response to smokers in the household.
Of this cohort, 152 children had their blood samples taken (of which 142 were able to be analysed for nutritional biomarker levels), and 145 children completed dietary recalls at baseline. There were 115 children who had their blood samples taken both initially and at the 12-month follow-up, while 120 children completed dietary recalls at both time points. There were no significant differences in F&V intake in those assessed only at baseline and those who underwent follow-up assessments (data not shown). Of the levels of nutritional biomarkers, only that of lutein–zeaxanthin was significantly lower among those who only provided a blood sample at baseline than among those who underwent follow-up assessments (t= − 2·4, P= 0·018).
In the Clarence Valley and the Nambucca Valley, 70 % of families (thirty of forty-three) collected 75% or more of available F&V boxes during the follow-up period. These data were not available for Coffs Harbour.
Impact of the fruit and vegetable programme on biomarker levels and fruit and vegetable intake
The median period between baseline and follow-up assessments was 370 d (interquartile range 354–407 d). In the overall sample of children, mean β-cryptoxanthin, lutein–zeaxanthin and vitamin C levels were significantly higher after participation in the F&V programme for 12 months than the baseline levels (Table 2). These changes remained significant after adjustment for sex, age and community (Table 2). There were no significant changes in α-tocopherol, γ-tocopherol or retinol levels. Adjustment for total cholesterol did not alter the mean change in the levels of carotenoids overall or in each community. Adjustment for the baseline level of biomarkers did not alter the mean changes in the levels carotenoids or vitamin C overall. There were no significant differences in biomarker levels by sex at baseline, although girls did show a greater mean change in β-cryptoxanthin levels than boys at follow-up (girls: 43·6 nmol/l; boys: 2·4 nmol/l, F= 3·43, P= 0·07). The use of vitamin and mineral supplements was reported by 13 % of the children at baseline and 9 % of the children at the follow-up assessments. The pattern of changes in biomarker levels was similar, except that the increase in β-cryptoxanthin level was not statistically significant, after excluding those participants who reported supplement use at follow-up.
Table 2 Plasma biomarker levels of the participating children (n 115) before and after the fruit and vegetable programme (Mean values and standard deviations; mean differences and 95 % confidence intervals)
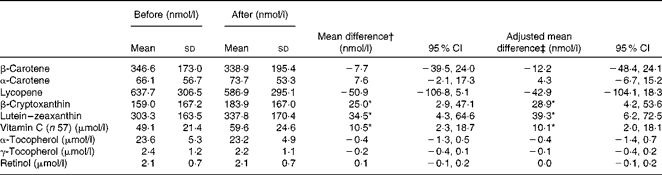
* Values were significantly different from zero (P< 0·05).
† Unadjusted mean difference (after–before), paired t test.
‡ Adjusted for sex, age and community using the general linear model (univariate ANOVA).
At baseline, the F&V programme participants (and/or carers) reported that the children's mean intake of fruit was 1·0 servings/d and that of vegetables (excluding deep-fried potato) was 0·8 servings/d in the 24 h dietary recalls, but their mean intake of fruit was 2·2 servings/d and that of vegetables was 2·5 servings/d in response to the short questions posed simultaneously (Table 3). There were no significant changes in F&V intake reported using 24 h dietary recalls for the overall sample in either the crude analysis (Table 3) or after adjustment for sex, age and community (Table 3). However, there was a small but statistically significant increase in fruit intake observed during the crude analysis, and a small, but statistically significant, decrease in vegetable intake was reported using short questions in the crude analysis (Table 3) and after adjusting for sex, age and community (Table 3).
Table 3 Fruit and vegetable intake of the participating children (n 121) before and after the fruit and vegetable programme (Mean values and standard deviations; mean differences and 95 % confidence intervals)

* Values were significantly different from zero (P< 0·05).
† Unadjusted mean difference (after–before), paired t test.
‡ Adjusted for sex, age and community using the general linear model (univariate ANOVA).
§ Questions about average servings of fruit and vegetables consumed, asked at the time of the 24 h dietary recall.
At follow-up, the participants or parents reported receiving their last F&V box 1 d to 4 weeks earlier. Analysis of these data showed that 100 children had received a box within the 7 d before the 24 h recall at follow-up, and of these, eighty-three had biomarkers at baseline and follow-up and eighty-six had 24 h dietary recalls at baseline and follow-up. On including only these data, no substantial differences were observed in the pattern of changes in the biomarker levels or self-reported F&V intake, except that the increases in β-cryptoxanthin and lutein–zeaxanthin levels were not statistically significant and the decrease in lycopene level was statistically significant after adjustment for age, sex and community.
On the whole, there were few differences of clinical import in response profiles between the communities, notwithstanding some statistically significant variations that were accounted for in multivariable-adjusted analyses of pooled data(Reference Black31).
Changes in macronutrient intake
There was a decrease in the self-reported macronutrient intake in the follow-up dietary recalls than at baseline, particularly of carbohydrates and total sugars (Supplementary file 2, available online). The mean total energy intake at baseline was 7570 (sd 3156) kJ, while at follow-up, it was 6482 (sd 2197) kJ. To facilitate comparison with the large variation in intake among these children aged 0–17 years, macronutrient intake per MJ was also analysed. In the analysis of macronutrient intake per MJ, there were small but statistically significant decreases in total sugar (1·6 g/MJ) and added sugar (1·2 g/MJ) intake, while the intake of other macronutrients was unchanged (Table 4). However, after adjustment for sex, age and community, there were no significant changes in the intake of any of the macronutrients per MJ overall (Table 4).
Table 4 Change in macronutrient density (g/MJ) among the participants aged 0–17 years (n 121) before and after the fruit and vegetable programme (Mean values and standard deviations; mean differences and 95 % confidence intervals)
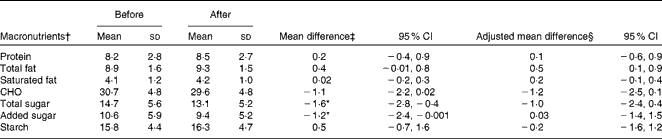
CHO, carbohydrate.
* Values were significantly different from zero (P< 0·05).
† Alcohol intake was not reported in any 24 h dietary recall.
‡ Unadjusted mean difference (after–before), paired t test.
§ Adjusted for sex, age and community using the general linear model (univariate ANOVA).
Discussion
The major findings of the present study of the impact of subsidised fresh F&V provided by three local Aboriginal community-controlled health services to disadvantaged Aboriginal families in rural communities were the significant increases in the levels of biomarkers of F&V intake including β-cryptoxanthin, vitamin C and lutein–zeaxanthin. In contrast to the changes in biomarker levels, there were no changes in the self-reported intake of F&V (when using either the 24 h dietary recall or the short dietary questions on usual intake). Furthermore, self-reported ‘usual intake’ was considerably higher than that reported in the 24 h dietary recalls.
These findings highlighted the well-known limitations of the self-reported dietary intake(Reference Bingham, Mann and Truswell32) and the role of biomarkers as alternative more objective measures of F&V intake(Reference Baldrick, Woodside and Elborn33). Collecting self-reported dietary intake data is particularly challenging for children, as the dietary intake data are collected with inputs from both a parent/carer and a child, which may lead to a different likelihood of bias due to difficulties with the estimation of quantities and under-reporting(Reference Livingstone, Robson and Wallace34). The decrease in self-reported energy intake at the 12-month follow-up (which would be expected to increase slightly in children) suggested that repeated self-reported dietary intake measurements may be susceptible to reporting bias in assessing the impact of nutrition interventions(Reference Natarajan, Pu and Fan35). The present study has also shown that it is feasible to obtain blood samples for the measurement of biomarker levels from children in a community-based intervention study with appropriate community engagement.
The baseline carotenoid levels in the present study were similar, except for lycopene level, which was higher, to those reported in a representative sample of 4231 American children aged 6–16 years(Reference Ford, Gillespie and Ballew36), and β-carotene level was also similar to that reported in 467 Australian preschool-aged children(Reference Karr, Mira and Causer37). While no comparable published data were obtained in Australian children, Hodge et al. (Reference Hodge, Cunningham and Maple-Brown38) found that Indigenous Australian adults have lower carotenoid levels than non-Indigenous Australians. These data are consistent with the finding that most children in high-income countries do not consume the recommended levels of F&V(Reference Heath and Panaretto39–Reference Lorson, Melgar-Quinonez and Taylor42).
The changes in vitamin C, β-cryptoxanthin and lutein–zeaxanthin levels were consistent with an increase in F&V intake. This pattern of changes suggests that fruit intake may have increased more than vegetable intake(Reference Baldrick, Woodside and Elborn33, Reference Dauchet, Peneau and Bertrais43, Reference Burri, Chang and Neidlinger44), although the self-reported data could not confirm this. Vitamin C level increased by 10 μmol/l (21 %). Khaw et al. (Reference Khaw, Bingham and Welch45) reported that each quintile of plasma vitamin C (10–20 μmol/l) was associated with a 20–50 g increase in fruit equivalent to half an apple or orange (and 10–20 g increase in vegetables) in a large prospective study in adults. The 18 % increase in β-cryptoxanthin level is consistent with an increased intake of orange/red F&V, particularly of citrus fruit(46). Lutein–zeaxanthin, the level of which increased by 11 %, is commonly found in green leafy vegetables, pumpkins, beans and maize, all of which are commonly included in communal meals consumed in contemporary Aboriginal communities(Reference Rowley, Su and Cincotta29). The level of β-carotene, which is widely distributed in many F&V, did not change in the present study. This may reflect the more limited bioavailability of β-carotene compared with the other carotenoids(Reference Burri, Chang and Neidlinger44, Reference van het Hof, Brouwer and West47).
The findings of the present study are consistent with a recent systematic review of the role of biomarkers in F&V intervention studies, which found significant increases in the levels of one or more biomarkers in thirty-five of the thirty-six F&V provision studies and fourteen of the sixteen nutrition counselling studies, most commonly in those of β-carotene, vitamin C, α-carotene and lutein(Reference Baldrick, Woodside and Elborn33). The proportional increases in β-cryptoxanthin, vitamin C and lutein–zeaxanthin levels observed in the present study were mostly greater than those reported in a nutrition counselling RCT(Reference John, Ziebland and Yudkin48) in which self-reported F&V intake increased by 1·4 servings/d but less than those in a food provision RCT in which the consumption of 500 g of F&V per d was compared with that of 100 g of F&V per d(Reference Broekmans, Klopping-Ketelaars and Schuurman49). Thus, carotenoids and vitamin C are responsive to changes in F&V intake(Reference Baldrick, Woodside and Elborn33), although further work is needed to confirm the linear dose–response relationship between F&V intake and the levels of these biomarkers, particularly of carotenoids.
Although the increases in carotenoid and vitamin C levels in the present study appear to be consistent with an increase in F&V of one to two servings/d, the self-reported dietary intake data do not confirm this. However, targeted F&V subsidies, as additions to national food subsidy programmes or as stand-alone interventions, have been shown to increase F&V intake by one to two servings/d or 20–30 % in women during pregnancy(Reference Burr, Trembeth and Jones50) and other life stages(Reference Herman, Harrison and Afifi51, Reference Kennedy, Champagne and Ryan52). There is limited evidence for families or children specifically. Increased intake of F&V of this magnitude is sufficient to improve long-term health outcomes, such as reducing the incidence of IHD or type 2 diabetes(Reference Crowe, Roddam and Key1, Reference Bazzano, Serdula and Liu3, 6, Reference He, Nowson and Lucas53, Reference Carter, Gray and Troughton54).
The key question is whether the short-term benefits of healthy food subsidies will translate to sustainable improvements in nutrition. There is evidence of healthier dietary intake or food purchases persisting, although attenuated, for 6 months after participation in food subsidy programmes(Reference Ni Mhurchu, Blakely and Jiang20, Reference Herman, Harrison and Afifi51). However, this was not noted in later follow-up of children whose families had previously participated in the Special Supplemental Nutrition Program for Women, Infants and Children in the USA(Reference Rush, Leighton and Sloan55). The evidence that price discounts are more effective at changing dietary behaviour than other strategies(Reference Ni Mhurchu, Blakely and Jiang20) and that price discounts or increases are projected to have a larger impact on low-income individuals or families(Reference Smed, Jensen and Denver17, Reference Powell and Chaloupka18) is important. Given the consistent association between less healthy dietary behaviour and lower socio-economic status(Reference Marmot, Friel and Bell56), it is likely that a range of complementary strategies will be needed to improve nutritional status among the most disadvantaged. It is unclear whether the nutritional challenges among Indigenous Australians who live in non-remote areas are different from those among other disadvantaged families in high-income countries. It is clear though that Indigenous Australians have much earlier onset and higher rates of chronic diseases(57) and thus have the most to gain from strategies that increase F&V intake.
An obvious limitation of the present study was the lack of a control group. This would have been difficult to implement given the clear economic benefit of the F&V subsidy, irrespective of any nutritional or health impacts that were the focus of the present study. However, a control group was contemplated as an equitable way to manage the waiting list of eligible families for this programme, but was ultimately considered impractical given the limited local research capacity in a busy Aboriginal community-controlled health service(Reference Sibthorpe, Bailie and Brady58). Regression to the mean and physiological changes with age was addressed in the analysis by including age and baseline levels as covariates in the model. Another limitation of the present study was that the impact of the F&V subsidies could not be separated from that of other elements of the programme, including nutrition education and increased engagement with Aboriginal primary health care services. However, other studies have suggested that the benefits of this programme are likely to be primarily due to the F&V subsidies(Reference Cobiac, Vos and Veerman14, Reference Ni Mhurchu, Blakely and Jiang20, Reference Burr, Trembeth and Jones50).
The use of non-fasting blood specimens was a potential source of error as serum vitamin C and α- and β-carotene levels have been shown to increase acutely several hours post-ingestion of carotene- or ascorbate-rich foods(Reference Jensen, Spiller and Pattison59, Reference Sánchez-Moreno, Cano and de Ancos60). However, it was considered impractical to expect these children to arrive fasted for these assessments. In addition, the biological half-life of the serum carotenoids ranges from 26 d for lycopene to 76 d for lutein(Reference Burri, Neidlinger and Clifford61), while the biological half-life of vitamin C is 10–20 d(Reference Naidu62), which suggests that the serum levels reflect intake in the previous several weeks, provided intake is relatively consistent. Thus, the significant increases in β-cryptoxanthin, lutein–zeaxanthin and vitamin C levels are likely to represent medium-term F&V intake, although the serum levels could have been influenced by recent ingestion of F&V, particularly vitamin C level by supplements or vitamin C-fortified foods.
The findings of this evaluation study of the nutritional improvements associated with F&V subsidies suggest that there is a need for a controlled study to verify these conclusions. Higher-quality longitudinal data would also provide the opportunity for an economic analysis that could also inform decisions about investing in food subsidies. The validity of biomarkers as indicators of F&V intake is well established in adults(Reference Baldrick, Woodside and Elborn33). International studies have established the normal range of plasma carotenoid concentrations in children(Reference Ford, Gillespie and Ballew36, Reference Karr, Mira and Causer37, Reference Brady, Lamb and Sokol63–Reference Malvy, Burtschy and Dostalova65). However, further studies are needed to confirm their validity and practicality as measures of F&V intake in interventions involving children. This is important given the discrepancies between self-reported dietary intake and biomarker levels observed in the present study.
The potential reduction in chronic disease risk due to increased F&V intake suggests that it would also be useful to assess the nutritional status of adults in family-focused nutrition intervention studies, given the long time lag between improving F&V intake in children and any manifestation of chronic diseases. Increased F&V intake is perhaps more likely to be sustained if it involves both adults and children in families. The sustainability of any change in F&V intake underpins any long-term health impacts. This programme provided a heavily subsidised supply of fresh F&V to these families, sufficient to meet the requirements of a family of five, which may be necessary to engage the most disadvantaged families. However, it would be useful to trial the impacts on dietary intake or nutritional status of F&V programmes that include lesser value subsidies or a larger participant contribution.
In conclusion, F&V subsidies improve the nutritional status of disadvantaged Indigenous Australian children. The measurement of plasma carotenoid and vitamin C levels as biomarkers are feasible to measure F&V intake in these children and may offer an objective alternative to self-reported dietary intake. Improving the F&V intake of these children is challenging and emphasises the need to link any subsidies with effective nutrition education to promote meal planning and preparation skills. The delivery of F&V subsidies in a community health setting strengthens the link between preventive health activities and improved nutrition. A controlled study of subsidised healthy foods is warranted to investigate the cost-effectiveness of this strategy to improve both the nutrition and health of the most disadvantaged families.
Supplementary material
To view supplementary material for this article, please visit http://dx.doi.org/10.1017/S0007114513001700
Acknowledgements
We acknowledge the families who participated in this evaluation study and the staff at the three health services, particularly David Ferguson and May Robinson from the Bulgarr fruit and vegetable committee, for the success of the F&V programme. Kerry Hampshire, Fiona Smith, Karen Spark, Nicole Turner, Lea Clayden and Marie Gough contributed significantly to the clinical assessments and data collection. Dr Karen Walker and Ms Jiang Hwa Lee at Monash University entered dietary recall data into FoodWorks and produced standard and supplementary nutrient and food intake analyses. The present study was supported by the National Health and Medical Research Council, Australia (PhD scholarship ID no. 520681 and program grant no. 320860). The authors' contributions are as follows: A. P. B., H. V., P. M. and K. O. designed the research; A. P. B. conducted the research; A. P. B., M. D., A. E., H. V. and K. O. analysed the data; A. P. B., H. V., P. M. and K. O. wrote the paper; A. P. B. had primary responsibility for the final content; C. S. K. undertook the analysis of carotenoids. All authors read and approved the final manuscript. None of the authors has a conflict of interest.