Instruments have long played an essential role both in the production of scientific knowledge and in the education of future scientists. While research and teaching are (and historically have been at least since the nineteenth century) very distinct activities, there are a number of experiments that cross the boundary between the research lab and the lecture hall, and are represented pedagogically in both concept and apparatus. However, in most cases, it was not the instrument itself, but instead the concept of the instrument that was materialized in the lecture hall in order to fulfil its purpose in the formation of future physicists.Footnote 1 As Ludwik Fleck notes in his discussion of initiating new members into a thought collective,
There is an apprenticeship period for … every field of knowledge, during which a purely authoritarian suggestion of ideas takes place, irreplaceable by a ‘general rational’ organization of ideas. The optimum system of a science, the ultimate organization of its principles, is completely incomprehensible to the novice …
Every didactic introduction is therefore literally a ‘leading into’ … The initiation into any thought style, which also includes the introduction to science, is epistemologically analogous to the initiations we know from ethnology and the history of civilization. Their effect is not merely formal. The Holy Ghost as it were descends upon the novice, who will now be able to see what has hitherto been invisible to him.Footnote 2
The process of initiation is supported in a very particular manner through demonstrations that can be described as materializations of historical experiments. These experiments can be labelled canonical; this means that the knowledge produced (or retrospectively connected) with these historical experiments is considered to be constitutive for concepts that are relevant to that branch of science.Footnote 3 With respect to these canonical experiments, I shall discuss in the following paper two broad categories of materialization that can be identified (particularly in the period from 1870 to 1920) when experimental concepts cross the boundary between research and teaching.
Instruments related to canonical experiments
When looking in the collections of physics instruments in lecture halls or in science museums, one finds several instruments that are related to some historical experiments. Apparatus labelled ‘Cavendish's gravitational balance’ or ‘Millikan's oil drop apparatus’, as well as the ‘Franck–Hertz experiment’, are examples that are still found in modern teaching collections, while the ice calorimeter according to Lavoisier and Laplace or Joule's experiment on the mechanical equivalent of heat are now only present in museums. But there is a category of apparatus, such as Coulomb torsion balances, that are both frequently found in museums and still present in today's teaching collections.Footnote 4 In fact, closer examination reveals that a substantial number of the historical instruments in museums’ collections were not actually meant to be used in research at all but served instead as teaching devices. So what was the relation between the concepts and practices associated with these pieces of pedagogical and research-experiment-based apparatus? In this paper, I will consider this general question with specific attention to a number of examples that date from the late nineteenth century and the early twentieth.Footnote 5 In doing so, I will argue that some educational set-ups were not suitable for the actual conduct of an experiment. While they strongly resemble experimental designs, and even though experimental practices can apparently be performed with them, they are materially unable to produce a reasonable result in terms of the respective theoretical concept. At the same time, there are a number of devices that were designed independently of the initial experimental set-up and were meant only as pedagogical demonstrations of the research context and content. These devices have been stabilized in such a way that these demonstrations cannot be characterized as experimental. In this paper, I will begin by discussing some general aspects of research experiments that are relevant for its scope. Subsequently I will use some examples of canonical experiments in order to show the strategies in transforming some canonical experiments into teaching demonstrations. In doing so, I will also discuss the meaning of these demonstrations for the formation of future physicists.
Distinguishing research and teaching instruments
In the cases I am discussing (but also on a general level), there are some requirements and limiting conditions that distinguish a research experiment from a teaching demonstration. First of all, research experiments took place in laboratories, while teaching demonstrations took place in lecture halls. While both spaces were designed to fulfil specific requirements, the requirements of these two spaces were not identical. Laboratories may have been designed as completely vibration-free, thermally stable or dark – all characteristics that could only be realized in a lecture hall to a limited extent. In turn, demonstrations had to take place within the constraints of the lecture timetable, which did not necessarily correspond to the chronological requirements of a research experiment.
Second, at least initially, experimental set-ups frequently possessed some flexibility. In the process of developing the final version of the experiment, adaptations were made that aimed at creating more stability and control. To illustrate this kind of development in a specific example, it is useful to look at the progression of the oil drop apparatus used by Millikan in determining what he called the ‘elementary electrical charge’. Millikan had started with water droplets in a high-voltage electrical field, which was modified by his PhD student Harvey Fletcher, who remembered decades later, ‘I decided to make a crude setup in the laboratory and try it before designing an elaborate one.’Footnote 6 Fletcher prepared two brass plates to serve as a capacitor, made a small hole in the upper plate and used an atomizer to spray some watch oil above that, so that small droplets entered the space between the capacitor's plates. He used an arc lamp for illumination and a cathetometer (a short-range telescope) to observe the light scattered by the drops. In the laboratory, batteries were connected to banks that could provide 1,000 V DC; Fletcher used one of these banks as well as a voltmeter. According to his account, within twenty-four hours he had set up his ‘crude apparatus’ and could confirm that this set-up works:
I saw beautiful stars in constant agitation. As soon as I turned on the switch [thus switching on the electrical field] some of them went slowly up and some went faster down. I was about to scream as I knew then that some were charged negatively and others positively. By switching the field off and on with the right timing one could keep a selected droplet in the field of view for a long time … I spent the rest of the day playing with these oil droplets and got a fairly reasonable value of e before the day ended.Footnote 7
There is, of course, an extensive history prior to Fletcher's work. But in subsequent years, Millikan and Fletcher (and other researchers) continued to develop the experiment, and their publications show that the apparatus, as well as practices with the apparatus, were evolving. The capacitor was inserted in a vessel, the vessel was inserted in a tank filled with oil (to increase thermal stability), the light was ‘cooled’ by filtering the infrared radiation, the air pressure in the vessel was controlled and could be manipulated, the humidity in the air was controlled, and so on and so forth. Importantly for the distinction between research experiments and teaching demonstrations, it was not only the experimental set-up that was under development. Crucially, experimental practice was also in flux, being developed through constant repetition: ‘In the interval between December, 1909, and May, 1910, Mr. Harvey Fletcher and myself took observations in this way upon hundreds of drops’.Footnote 8
The important aspect for the discussion in this paper is that initially the experimental set-up (and also the procedures associated with it) were very flexible and variable. In the course of the experimental development, an essential objective of the research of Fletcher, Millikan and their colleagues was to establish more stability and control over the experimental space – the experimental space in this example being the space between the capacitor plates in which the oil droplets move. However, this stability is not simply materialized, but results from an interaction of the experimenters with the apparatus that aims at controlling the experimental space, and thus results in stability. Thus the experimental set-up does not provide stability in and of itself, but only through interaction with specific experimenters – in this case Fletcher and Millikan, who had taken the effort to develop the necessary skills and the tacit knowledge. In this process, the experimenter's regress, as discussed by Collins, is relevant: stability is taken to be achieved through the ability to produce reliable and ‘correct’ results.Footnote 9
This is obviously different for teaching-demonstration devices: these instruments have to act in a stable and reproducible manner in order to produce textbook-consistent results. However, this stability is not the outcome of a stabilization process by the user. Instead this has already been secured by the manufacturer and is materialized in the instrument. The stability of the teaching device is therefore – in contrast to the research instrument – largely decoupled from the user. Consequently, the stability of the demonstrations is independent of the user, at least as long as the user has some competence in teaching demonstrations. Thus there are distinct requirements for instruments that are meant to be used in teaching, and as a result, when an experiment and the related set-up are transformed into a teaching demonstration, these different requirements must be met through instrumental design.
In a previous paper, I discussed the transformation of experimental instruments into teaching devices.Footnote 10 In this respect, I claimed that there are three aspects to this process: downscaling and simplifying research instruments, stabilizing the experiment, and the emergence of instruments that become iconic objects in the lecture room.Footnote 11 In this paper, I will examine two further elements in this process. The first relates to the presentation of iconic objects that served as a representation of either an experiment or the actions associated with an experiment. These objects are an integral part of a retrospective narration that is told in the lecture theatre and by the textbooks, connecting the establishment of central concepts (such as energy conservation) to certain canonical experiments (such as Joule's experiments of the mechanical equivalent of heat). As I will show in the course of this paper, this leads in some cases to the use of iconic objects for teaching purposes. The second aspect relates to the development of educational devices that served to produce the representation of a result of the same canonical experiment. By contrasting the experiment itself with the subsequent teaching devices that were marketed at the end of the nineteenth century and in the early twentieth, some key peculiarities of educational demonstrations will become apparent.
James Prescott Joule and the mechanical equivalent of heat
My first example in this paper is the determination of the mechanical equivalent of heat by James Prescott Joule – the central outcome of his research is a paper published in 1850.Footnote 12 In this paper, he described the paddle wheel experiment in which he demonstrated the equivalent convertibility of mechanical force into heat. Inappropriately simplified, the experiment can be described as a huge water stirrer operated by sinking weights (Figure 1). Crucial are two measurements – the distance of the weights’ fall and the increase of the water's temperature. Obviously, things are not that easy; this is evident from the fact that Joule spent several years working on his measurements, publishing preliminary results in 1845 and 1848, and prior to working with the paddle wheel, had used different experimental set-ups to calculate the mechanical equivalent of heat. Interestingly, referring to the results of Joule's experiments, John Tyndall stated retrospectively, ‘As the experience of the experimenter increased, we find that the coincidence of his results becomes closer’.Footnote 13

Figure 1. Joule's paddle wheel. Inside the vessel in the middle is the paddle that stirs the water. J. P. Joule, Das mechanische Wärmeäquivalent (tr. W. Spengel), Braunschweig: Vieweg, 1872, with permission of the Landesbibliothek Oldenburg.
When analysing the experiment, several aspects turned out to be crucial: one relevant detail was the sensitivity of the thermometers as well as Joule's capability of reading them with great accuracy. Another aspect was the room – it had to have a high heat capacity in order to minimize the change in the room's temperature during the experiment. In order to have an effect that can be measured with the necessary accuracy, the temperature increase had to be high enough – consequently, the weights were as heavy as twenty-six kilograms in total, and fell a distance of some 1,250 inches (almost thirty-two metres) – this was possible by dropping the weights twenty times and winding them up in between. However, this had to be simultaneously done quickly and in a very controlled manner – Joule did not mention who actually carried out this work, but clearly this unknown helper was as crucial to the success of the experiment as Joule himself, the room or the thermometers.Footnote 14
As noted, these requirements were not met immediately; Joule spent years establishing the stability and control necessary to produce a result he could claim to be reliable. Thus the experiment can be described as a process, rather than an event. In this respect, the aim was not only to produce a particular value in this process, but to produce stability and exert control with respect to the behaviour of the set-up. Both aspects are reflected in the resulting data that Joule published in his final paper – actually not the absolute data in comparison to the value accepted nowadays, but the standard deviation of the forty measurements: the mean resulting increase of the temperature was 0.56 °F with a standard deviation of 0.010 °F. Perhaps even more relevant with respect to my argumentation is the height the weights fell in each of the trials. This value has a standard deviation of 0.3 per cent, which can be seen as an indication of the control that was achieved in the process of winding up the weights by an (unmentioned) assistant.
In his publication, Joule explicitly described a number of measures with which he wanted to increase the reliability of his result. Most prominent are control measurements which serve as a correction for thermal radiation effects. In preliminary experiments that are mentioned in the paper, Joule determined the necessary correction for friction effects and for the speed with which the masses touch down on the ground. Other aspects that turned out to be necessary through the analysis with the replication method are only implicitly visible – for example, the temperature of the water has to be in a range that does not differ too much from the room temperature.
The process of creating the final version of the experiment required modifications of both the apparatus and the procedures – thus the flexibility and openness of the initial experiment enabled the developments that led to the experiment's final version. In this respect, Joule's experiment shows characteristics that are comparable to that formulated for Fletcher and Millikan's experiment. Both experiments required time to create the necessary stability and to enable the experimenter to achieve control of the experimental behaviour. In both, experimental behaviour is not limited to the performance of the apparatus itself, but also includes the experimenters’ actions. These actions had to be carried out in a particular manner, and also required control and stabilization. All these qualities were necessary in order to finish the experimental process.
Performative teaching: Max Kohl's paddle wheel apparatus
These characteristics of Joule's experiment become relevant in comparison to the various educational devices that were marketed with respect to the experiment. A remarkable example is the instrument sold by Max Kohl, one of the most prominent makers of demonstration instruments for physics teaching in the late nineteenth century and the early twentieth. This is actually a paddle wheel apparatus that looks – at first glance – very much in accordance with Joule's apparatus (see Figure 2).Footnote 15 When seeing the instrument, but even more when watching a demonstration, the optical resemblance between the (mental image of the) research experiment and the teaching demonstration (which has been re-created by Paolo Brenni, and can be seen in one of his online videos) is striking, and – this is part of my argument – intentional. The instrument-maker (Max Kohl) obviously aimed at enabling the demonstrator to create a vivid image in the minds of their audience of how Joule had actually carried out the experiment.Footnote 16
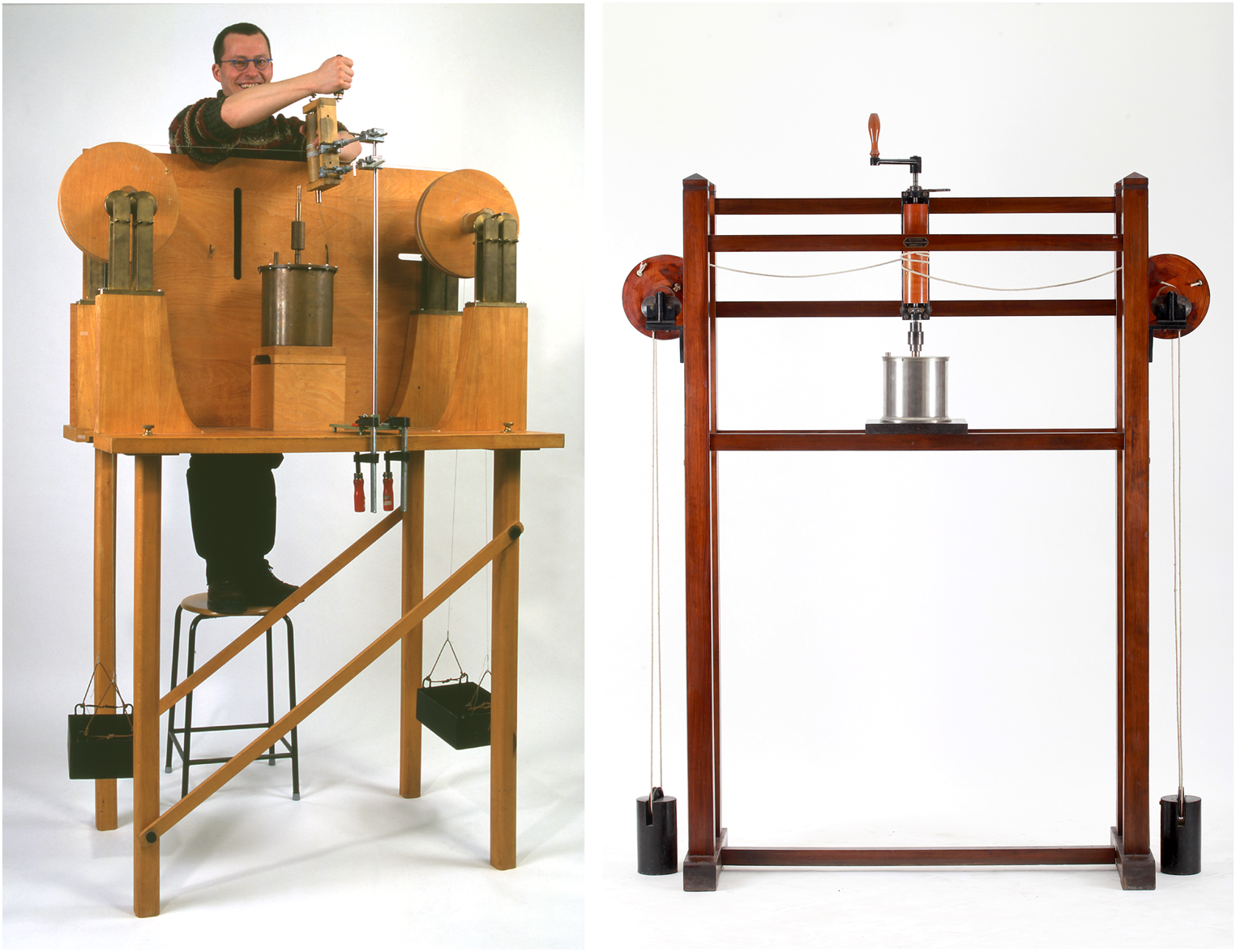
Figure 2. Left: reconstruction of the paddle wheel used in the analysis with the replication method, with permission of the Carl von Ossietzky Universität Oldenburg. Right: teaching version of the paddle wheel apparatus from the company of Max Kohl, with permission of the Fondazione Scienza e Tecnica, Florence.
However, comparing the two set-ups more closely, significant differences become evident. The weights are not the same: Joule used about twenty-six kilograms, while the educational set-up uses only about twelve kilograms. The amount of water in the vessel is only slightly reduced in the educational version (six litres versus five litres), thus the increase in temperature will be significantly smaller unless the height of the fall of the weights is increased considerably. Also, the design of the paddles is different; as a result, friction is apparently less, which is evident from the higher sinking rate of the weights in the educational set-up. Two other changes affect the operation of the device: the demonstrator connects the roller and the axis of the paddle wheel through the operation of a metal lever, and the thermometer remains in the vessel during the entire operation.
This latter modification appears to be only marginal, but it has some relevant consequences. When the thermometer remains in the vessel, it is in permanent thermal equilibrium with the water. In Joule's experiment, the thermometer is outside the water, meaning that thermal equilibrium has to be restored. Normally, this means that one has to wait until the instrument reads a constant temperature. However, in Joule's experiment the thermometers are very sensitive – as Joule claimed, they can be read to 1/200th of a degree Fahrenheit – and readings are not stable at this level of accuracy. Thus the operator has to have a profound understanding of the nature of the temperature measurement in order to make an adequate decision about when to read the temperature. In contrast, in the educational version the temperature can be read as soon as the operation comes to an end, or even before. Therefore it appears that measurement with the educational device is much easier.
Yet there are problems which make it unlikely that the device could ever have produced data. As pointed out, the heat capacity of the room was one of the crucial details in Joule's experiment (the cellar of the family brewery) – this is certainly different to a lecture hall full of students where the required uniformity of temperature could not be secured. Moreover, the weights are smaller, so in order to achieve the same mechanical energy, a larger height is required – this can be realized by winding up the weights and letting them fall again not just twenty times, but perhaps as many as forty. Joule stated that one run took him about thirty-five minutes – winding up the weights twice as often will obviously take even more time. Thus both technical and practical arguments make it questionable that the teaching device was able to produce data that could be used to calculate the mechanical equivalent of heat within the context of the lecture itself. Additionally, as Brenni's reconstruction shows, the demonstration in practice is noisy, and could not realistically have been carried out alongside a lecture in progress for any length of time. Consequently, the actual performance of the experiment was virtually impossible: the device was not there to produce data.
If producing data for demonstration was not the purpose of the device, one may ask, what could be done with it instead? On the one hand, the apparatus is clearly iconic, modelling a research experiment that is considered fundamental to the development of physics. Yet it is not meant just to be looked at, but to be operated. In my understanding, this device was meant to demonstrate not just the apparatus of Joule's experiment, but also the procedures surrounding them. Thus demonstrating this apparatus in a lecture enabled the students to develop an understanding of the instrumental as well as procedural aspects of Joule's experiment – winding up the weights, reading the heights of the weights, reading temperatures. Certainly, one can question whether this approach could have resulted in an appropriate understanding of the procedures; particularly reading thermometers with the necessary accuracy might have been more challenging than these aspiring physicists, familiar only with thermometers that can be read to one degree, imagined.
But the point here is that the demonstration puts students in a situation somewhat comparable to the (virtual) witness that was common in the latter half of the eighteenth century, enabling them to prove the trustworthiness of experimental results. As Golinski points out, ‘printed texts that were deliberately crafted to place the reader in the position of “virtual witness” to experimental demonstrations’ were characteristic of eighteenth-century knowledge production and communication.Footnote 17 Both situations enabled their audiences to generate a mental image of the actual experiment. Just as with reading text, witnessing the teaching demonstration required the audience to undertake an exercise in mental imagination and accommodation, since the demonstration itself did not correspond to the original historical experiment, but was subject to a (at least in part intentional) distortion.
It also, of course, involved a more insidious epistemological form of distortion with respect to the image of experimentation that it created: the idea that one had only to place an apparatus in a room (actually in any room) and do some manipulation in order to produce science. As noted earlier, Joule's experiment was developed over the course of some five years, and crucial aspects of this development were the initial openness and flexibility (metaphorically speaking) of his experiment. In contrast, the teaching device is simple, straightforward and fixed, with no flexibility with respect to the instrument or its operation and little skill needed on the part of the demonstrator. But because it is operational, providing the audience with an imitation of Joule's experimental procedures, it becomes not just an iconic experiment but a didactical demonstration of what it meant to do science. Notably, this was not the only way in which the mechanical equivalent of heat was to be taught. Other teaching devices address other aspects of Joule's experiment – in particular the actual value that emerged. In the next section, two of these demonstrations are discussed in detail.
Product-oriented teaching: Puluj's and Callendar's instruments for teaching the mechanical equivalent of heat
Some decades prior to the paddle wheel teaching demonstration, Johann Puluj proposed a completely different apparatus related to teaching the mechanical equivalent of heat.Footnote 18 Central in his instrument were two conical metal cups, one of which fitted exactly into the other. The inner cup was filled with mercury and a thermometer was inserted, while the outer cup was attached to a disc that could be rotated from a hand-operated wheel. A string connected the inner cup to a scale pan in which a few grams of lead were placed. When the apparatus was set in motion, the outer cup rotated at a certain speed, causing friction between the two cups. The frictional force made the inner cup move slightly, and as a result the scale pan was lifted. The speed of the outer cup was adjusted so that the scale pan hovered at a certain height (this corresponds to a dynamometrical brake). Consequently, frictional force and gravitational force balanced each other, and from the number of rotations the mechanical energy could be determined and set in relation to the increase of temperature – as a result, the value of the mechanical equivalent of heat can be determined (Paolo Brenni has demonstrated this apparatus; see also Figure 3).Footnote 19 In his 1876 publication, Puluj points out that he used this device for demonstrations in his lectures. The result, he reported,
is in best agreement with the number 424,9 found by Joule as the mean of a large number of very well corresponding values. This result can also be taken as a measure of the accuracy with which the demonstration [Versuch] can be carried out with the help of this simple apparatus.Footnote 20
Obviously, this teaching device is related to Joule's work only by topic. Both deal with the mechanical equivalent of heat, but Puluj's apparatus is not related to Joule's, it has no historical context, and the only thing one can do is turn the handle and produce temperature readings in relation to the number of revolutions – and these readings, together with the mass of the small lead balls, result in the mechanical equivalent of heat. Consequently, the quality of the instrument was judged by its ability to produce a value that corresponds to the one found in the textbooks (or the one published by Joule).
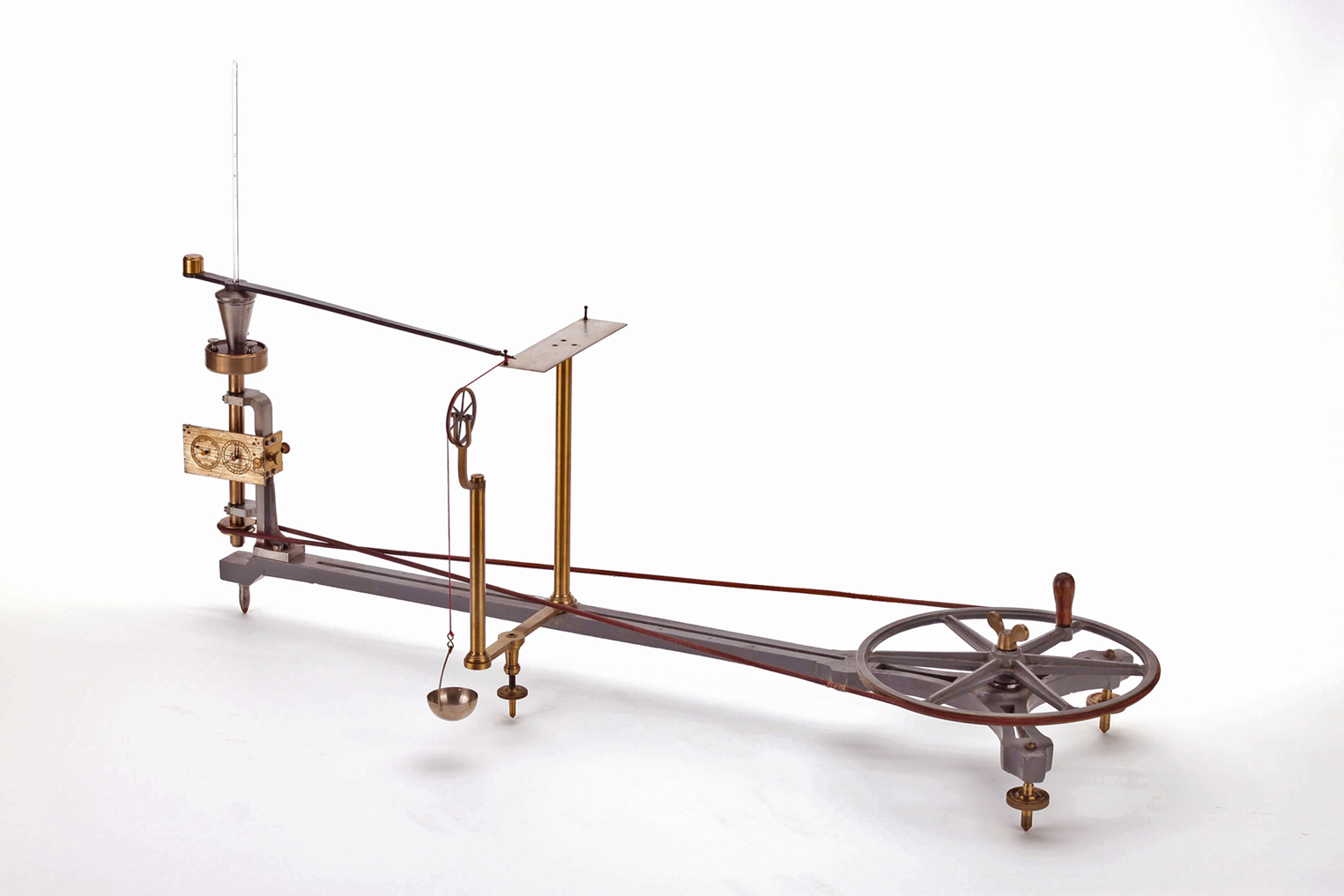
Figure 3. Puluj's apparatus for demonstrating the value of the mechanical equivalent of heat, with permission of the Fondazione Scienza e Tecnica, Florence.
Like Puluj, Hugh Longbourne Callendar proposed a set-up that was meant to be used in teaching the mechanical equivalent of heat by demonstrating the ability to produce the value that was connected with Joule's experiments. Central to Callendar's device was a ‘metal cylinder containing liquid [which] is mounted so as to be capable of rotation about a horizontal axis. A belt passing over the circumference of the cylinder carries unequal weights on either side’.Footnote 21 In the demonstration, the cylinder was rotated at a velocity that made the weights levitate – consequently, the friction compensated the difference between the gravitational forces and was thus known. A counter registered the number of revolutions; as a result, the mechanical energy was determined. A thermometer was inserted in the cylinder to determine the heat and, in comparing these two values, the value of the mechanical equivalent of heat was demonstrated.Footnote 22 As in the case of Puluj's apparatus, Callendar's device also produced a value that corresponded to the established value for the mechanical equivalent of heat.
In the case of both devices, there is just one way in which each can be operated. Unlike the paddle wheel demonstration device, limited skill is needed in order to produce proper results, and there are hardly any options for modification of the apparatus. If operated correctly, both instruments produce one particular number: failure to produce that number demonstrates the operator's incompetence, as opposed to a problem with the machine or the concept.Footnote 23 At the same time, the measurement does not take much time. Puluj reported in his paper six series of measurements, each containing between two and ten measurements, each of which took less than a minute, making his claim that this device was suitable for demonstrations in his lectures seem reasonable. Experimentation with one of Callendar's devices in the collection of the HistoLab at the Europa-Universität Flensburg showed that it was likewise possible to carry out demonstrations in a similar amount of time (see Figure 4).Footnote 24 This experience corresponds to the claims made by the Max Kohl A.G. catalogue, which stated explicitly for the electric motor version, ‘The lecturer can obtain values accurate to approx. ½% in the presence of the audience within 10 minutes’.Footnote 25 However, neither of these instruments could do anything but produce the textbook value within margins of error, meaning that ‘nature’ could not act other than to respond by producing the textbook value. As a result, the interaction of the demonstrator with the device resulted in a predetermined result – there was no flexibility in either the procedures or the outcome.
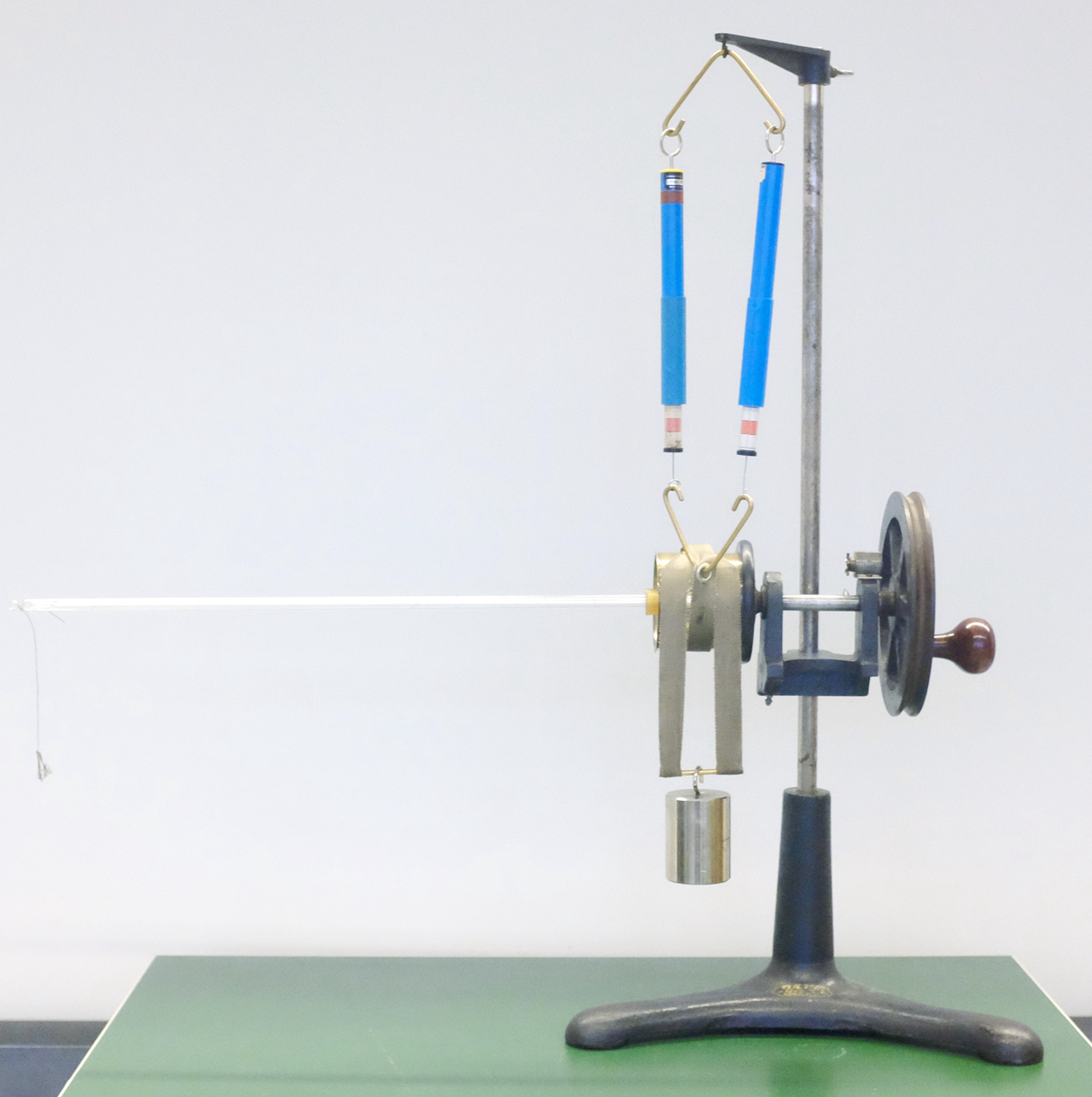
Figure 4. Callendar's apparatus for demonstrating the value of the mechanical equivalent of heat. Photograph Ruben Holländer, Europa-Universität Flensburg.
This can be interpreted in two ways. On the one hand, the lecturer established the veracity of the scientific knowledge that he is teaching – there are no doubts; there is no uncertainty. He is possessed of clear pedagogical authority, relevant with respect to the initiation of the students into the thought collective to which the lecturer belongs. Demonstrations which (however contrived) enable students to witness with their own eyes how, and according to which rules, nature works can be even more powerfully convincing than lecturers and textbooks.Footnote 26 Yet there is another level of significance here: the demonstration also shows the lecturer's (and by extension the collective's) authority over nature. Demonstrations and experiments (which cannot necessarily be distinguished by the students) are designed in such a manner that nature has to respond – and the response is already predicted by science (or the lecturer). This encapsulates the classical understanding of knowledge production through experiments (or demonstrations in teaching). See, for example, Brockhaus's Conversations-Lexikon from 1847, under the entry ‘Versuch oder Experiment’:
any observation in the natural sciences that is made under conditions that one has intentionally arranged to exclude certain influences, to add others and thereby to quasi-enforce nature to answer about the essential and non-essentials of a phenomenon.Footnote 27
Through these teaching demonstrations, nature was exhibited and perceived as a passive actor in the lecture hall that had, and could if necessary be forced, to answer. At the same time, the lecturer, representing the thought collective of physicists, was the one who could make nature reveal the truth. At this time (we are at the beginning of the twentieth century) lecture halls were still single-sex spaces, populated by male lecturers, demonstrators and students. In this respect, Friedrich Kohlrausch's recollection of his experience of the late nineteenth-century lecture experience is remarkable. As he pointed out in the introduction to the eleventh edition of his Lehrbuch der praktischen Physik,
it is justified to look back to the time when the book was written [1870]; but it is more interesting to focus, instead of on the book, on the conditions existing at that time, all the more so because the number of colleagues who know that situation from their own experience has become small. For them, too … an abstraction will be necessary if they want to visualize again the narrow patriarchal condition which existed half a century ago in the operation of the physical institutes.Footnote 28
Even though Kohlrausch certainly did not have any gender issues in mind when he used the term ‘engen patriachalischen Zustand’ in his introduction, it is helpful to understand it also in this respect. This is even more the case when Carolyn Merchant's interpretation is added to the picture: ‘Merchant rightly called attention to the adamant gendering of nature as female in both ancient and modern science traditions.’Footnote 29 To put it bluntly, what we have in the late nineteenth century and the early twentieth (and this is not necessarily limited to this period) physics lecture hall is a male expert (the lecturer, possibly supported by a male demonstrator) and male novices who are to become members of a collective that is (almost) entirely male. The only actor in the room that is perceived as female (following Merchant's interpretation) is nature, but nature is not free to act, but is forced in the demonstration to reveal the truths that the lecturer claims to know.Footnote 30
This understanding shows that the lecture hall was a gendered place, and that teaching demonstrations played a significant role in establishing the credibility of the (male) scientist who apparently controls nature and demonstrates the ability to make nature reveal the truth. While this is true with respect to all demonstrations made during a lecture, the focus of this paper is on representations of canonical experiments. So what was their significance in this context?
These representations treat certain concepts as fundamental, even if the research experiments initially were not directly connected to these concepts. Thus in some cases the teaching version of the canonical experiment was used to demonstrate something different to the result of the initial experiment. In these cases, we have another transformation: alongside changes in the set-up used, there is also a conceptual transformation in what is identified as the result. This becomes evident when looking at some of the examples mentioned in the introduction: Joule's work became related to the principle of energy conservation, Cavendish's experiments were read in terms of establishing the gravitational constant, Coulomb's work was related to Maxwell's equations which are fundamental to electrodynamics.Footnote 31 However, some historical experiments were taught in their original research context: Millikan's oil drop experiment was connected to the establishment of an atomic structure in electricity, and the ice calorimeter was crucial for the concept of heat. For either category, the overall message of all the teaching representations of historical experiments was clear: fundamental scientific concepts are based on empirical evidence and can thus be regarded as true.
To summarize the discussion so far: with respect to the example of teaching the mechanical equivalent of heat, there are two different types of teaching device that addressed different aspects of Joule's experiment. The paddle wheel demonstration device focused on the experimental procedure, while Puluj's and Callendar's devices prioritized the experimental results.Footnote 32 Yet, despite the differences, there were also similarities. Most notably, all instruments were stabilized in a manner that made them fairly easy to operate while minimizing flexibility. This makes sense, since teaching instruments were meant to be used in lectures where the time available for demonstration was limited. Both sets of devices could be used relatively quickly, the paddle wheel to showcase the performance of experimental practice, while the other devices produced the experimental values that were also found in the textbooks.
Expanding the case study
These aspects discussed so far are not unique with respect to Joule's experiment. For example, in the late eighteenth century, the French military engineer Charles August Coulomb carried out electrical experiments and established the torsion balance as a device for demonstrating the force distance relation for electrical charges. The torsion balance became a central object in the study of electricity, and, unsurprisingly, soon the instrument also entered the lecture hall. However, it also soon proved problematic. Paul Louis Simon in Berlin complained in a letter published in the Annalen der Physik that he intended to ‘dispense with Coulomb's compound torsion apparatus, which is all too unsteady for use in lectures’.Footnote 33 Some twenty years later, Georg Simon Ohm noted in his treatise on electrical conductivity that he had his own device made on site for his experiments, as the two existing torsion balances were not at all usable for accurate experiments.Footnote 34
The criticisms formulated here can be interpreted in various ways. On the one hand, the torsion balances may have been devices for research that were less stabilized and consequently demanded higher skill from the users because of the lack of stabilization. Simon's criticism in particular can be read in this direction. On the other hand, Ohm at least seems to have come across torsion balances in the collection that were not very suitable for his research purposes. Of course, these instruments may also initially have been designed for research, but may have been of low quality. This would not necessarily mean that they were not suitable for teaching: a poor research instrument could still be used to demonstrate the actions that were to be performed in order to produce the experimental result. But the instrument Ohm had in the teaching collection may also have been designed specifically for teaching purposes. This would imply that there was little possibility of adapting instruments stabilized for lecture purposes to a research question. Again, then, while a highly stable instrument can imitate experimental actions (similar to Max Kohl's paddle wheel apparatus) in order to illustrate the use of the apparatus in question, it cannot actually produce data. How this performance is perceived by the students would then depend on the context given in the lecture. They can see it as a teaching demonstration of how a historical experiment was carried out in principle, but they can also perceive it as a demonstration of how experimental measurements are actually carried out.
However, other teaching examples of the torsion balance exist. One example can be found in the historical collection of the Department of Physics of the Norges Tekniske Høgskole, now NTNU, in Trondheim. Here, a torsion balance, again sold by Max Kohl, was used in lecture demonstrations between 1910 and the early 1920s (Figure 5).Footnote 35 Close examination reveals that this instrument shares key characteristics with the demonstration of the mechanical equivalent of heat provided by Max Kohl. The torsion micrometer was made out of a single piece of brass with the scale only indicated, and did not allow for any quantitative measurements. Consequently, the device can be used for demonstrations of the procedures but not for the production of data.Footnote 36 Even in the late twentieth century, we find teaching devices that are labelled torsion balance, but apart from the measuring principle have little in common with Coulomb's instrument. These set-ups have a bifilar suspension, significantly larger conducting spheres replace the pith balls in Coulomb's instrument, and they use a light beam reflected from a mirror that is attached to the upper end of the holder which carries the movable ball. The spheres are charged with either a rubbed plastic rod or a high-voltage power supply. Then respective measurements are carried out that demonstrate the force being proportional to the product of the charges and inversely proportional to the square of the distance between the centre of the spheres.Footnote 37

Figure 5. Set-up of demonstration apparatus for the physics lecture on electrostatics at the Norges Tekniske Høyskole (Norwegian Institute of Technology), 1915. Statsarkivet i Trondheim, A-2831 – Norges Tekniske Høgskole (NTH) Fysisk institutt/fysikkseksjonen, Ua: 1 Negativer fra oppsett til demonstrasjonseksperimentene er en fotodokumentasjon av demonstrasjonseksperimenter i fysikkforelesningen fra 1914/15.
What remains slightly unclear in comparison with Joule's and Coulomb's research experiments is the question of the development of the instrument and the procedures over time. At first glance, Coulomb used the torsion balance to make the electrical experiments, and came up with the inverse square law immediately after demonstrating the law of torsion for metal wires in 1784. Thus it seems that the stabilization process that could be identified both in Joule's experiments and in the collaboration between Fletcher and Millikan did not take place. However, Coulomb did carry out a research programme focused on the mathematical description of lightning rods and their behaviour – and in the course of this study (which took from 1785 until 1791) it is possible to see the development of both the instrument and the procedures for its use.Footnote 38 This case would be another example for both the stabilization process carried out by the experimenter and the modification of the transformation process that was necessary for crossing the boundary between research and teaching.
Conclusion
Teaching demonstrations that are related to canonical experiments have various shared characteristics. Some examples include downscaling and simplifying research instruments, or stabilization of the demonstration devices that reduced the skills required of the demonstrator, while some devices served as significant iconic objects in the lecture room. Particularly for the period analysed in this paper (1870–1920), one aspect is common to the teaching devices deployed there – they have become stabilized in that the results which they produce are entirely predictable. This stability is based on and in their material design, which makes it extremely difficult, if not impossible, for them to behave in a way other than that expected by the lecturer.
However, teaching devices in the nineteenth century and the early twentieth, particularly those used to demonstrate canonical experiments, also shared other key characteristics. In some cases, it was the procedures of the canonical experiment that were pedagogically significant: in these examples, it was not the outcome of the experiment that mattered, especially where the instrument was actually unable to produce straightforward data. Such data could be produced by alternative, complementary, experimental set-ups – although these would also need to be stabilized, in that the operator should be fairly easily able to produce data that correspond to the result of the historical textbook experiment, be that a value or a relation. It was, however, crucial to reduce the need for skill on the part of the operator: pragmatically, no lecturer or demonstrator would have the time or resources necessary to acquire all the tacit knowledge required for the successful manipulation of historical research apparatus. Instead, the device had to materialize the working principle at stake in such in a manner that the demonstrator would easily be able to produce the expected outcome.Footnote 39
In this respect, one can ask, what was the purpose of teaching demonstrations, particularly those demonstrations related to canonical experiments? Two elements are particularly important here. On the one hand, the inclusion of these experiments in the curriculum helped to create a narrative in terms of disciplinary tradition: physicists-to-be are brought into direct connection with the historical heroes of the past. This would also explain why these teaching demonstrations were placed in the context of a creation of scientific identity or persona. As Daston and Sibum observed, ‘identities and the names that designate them are recycled from generation to generation, so that ancestors are endlessly reincarnated and the social structure of the clan appears static’.Footnote 40 The cases discussed here demonstrate that this applies not just to individuals, but also to experiments. These experiments from the history of physics became (in the lecture, and thus in the formation of future physicists) decontextualized and timeless; their outcome was communicated as true in an absolute sense. On the other hand, the lecturer's performance also demonstrated his ability to make nature perform according to scientific laws, when their apparatus produced the expected values (product-oriented), or when their practice enacted the procedures whereby nature's secrets were unveiled by historical actors (process-oriented). Both types of demonstration enacted the control and dominance of the lecturer over nature – either way, nature could not behave otherwise than as the lecturer, reflecting the established textbook knowledge, had specified. Canonical experiments are particularly significant here as the respective knowledge and its production became an integral part of the identity of the discipline.
Acknowledgements
I am indebted to Roland Wittje, David Pantalony and in particular the late Christian Forstner, who encouraged me to develop my initial thoughts about the topics of this paper into a coherent argument. I am also grateful to two anonymous reviewers and to Amanda Rees who helped me to improve the clarity of my argument through their constructive and appreciative suggestions.