Introduction
Introduction of non-indigenous species is a serious threat to native biodiversity and a major source of biological pollution (Carlton, Reference Carlton1989; Boudreaux and Walters, Reference Boudreaux and Walters2006; Paul and Kar, Reference Paul and Kar2016; Soares et al., Reference Soares, de Lima Xavier, Dias, da Silva, de Lima, Barroso and Bandeira2022). Many invasive gelatinous zooplankton species have considerable ecological and economic effects on the recipient ecosystem (Galil et al., Reference Galil, Spanier and Ferguson1990; Purcell et al., Reference Purcell, Shiganova, Decker and Houde2001). Well-known examples include Mnemiopsis leidyi in the Black Sea, Blackfordia virginica on the Atlantic coast of Portugal, and Rhopilema nomadica blooms in the Mediterranean Sea (Brodeur et al., Reference Brodeur, Sugisaki and Hunt2002; Galil, Reference Galil2007; Teodosio et al., Reference Teodosio, Leitão, Range, Gutierrez, Morales, Morais and Chícharo2009; Galil, Reference Galil2010; Costello et al., Reference Costello, Bayha, Mianzan, Shiganova and Purcell2012). However, many invasions of gelatinous zooplankton species go unrecognized. This may be due to cryptic morphologies, morphological heterogeneity due to phenotypic plasticity or rapid adaptive evolutionary divergence, or a lack of obvious impact on native species assemblages (Bayha and Graham, Reference Bayha and Graham2014). Moreover, non-indigenous species are sometimes misinterpreted as native species due to morphological similarity with native species or insufficient baseline knowledge of the native ecosystem (Thiel, Reference Thiel1935; Miglietta and Lessios, Reference Miglietta and Lessios2009). The hydrozoans are one of the most common and diverse groups of gelatinous plankton, yet many of them are inconspicuous invaders (Graham and Bayha, Reference Graham, Bayha and Nentwig2008). DNA barcoding has been valuable for identifying their presence (Holland, Reference Holland2000; Holland et al., Reference Holland, Dawson, Crow and Hofmann2004). An example of an enigmatic hydrozoan species with a complex invasion history is Gonionemus sp., which is native to the northwest Pacific, where it is notorious for causing painful stings to humans. Gonionemus sp. is introduced in other parts of the world (Rodriguez et al., Reference Rodriguez, Pujol, Mianzan and Genzano2014; Gaynor et al., Reference Gaynor, Bologna, Restaino and Barry2016; Govindarajan and Carman, Reference Govindarajan and Carman2016; Govindarajan et al., Reference Govindarajan, Carman, Khaidarov, Semenchenko and Wares2017, Reference Govindarajan, Källström, Selander, Östman and Dahlgren2019), but identification is sometimes challenging due to its similarity to the less toxic, closely related Gonionemus vertens lineage (Govindarajan and Carman, Reference Govindarajan and Carman2016). Some other globally invasive hydrozoans are B. virginica (Thiel, Reference Thiel1935; Mills and Sommer, Reference Mills and Sommer1995; Wintzer et al., Reference Wintzer, Meek and Moyle2013; Freire et al., Reference Freire, Genzano, Neumann-Leitão and Pérez2014), Maeotias marginata (Mills and Sommer, Reference Mills and Sommer1995; Mills and Rees, Reference Mills and Rees2000), Turritopsis dohrnii (Miglietta and Lessios, Reference Miglietta and Lessios2009), and Craspedacusta sowerbii (Jankowski et al., Reference Jankowski, Collins and Campbell2007).
Here, we describe the existence of a new non-indigenous hydromedusae species Vallentinia gabriellae Vannucci Mendes, 1948 from the Cochin estuary on the coast of Kerala, India. Until now, this species has only been recorded from tropical Atlantic waters (Kramp, Reference Kramp1959; Krumholz, Reference Krumholz1963; Lytle, Reference Lytle1964; Foster, Reference Foster1973; Rey et al., Reference Rey, Kain and De Freese1992; Ahuatzin-Hernández et al., Reference Ahuatzin-Hernández, Canul-Cabrera and Eúan-Canul2020). We conducted an integrative taxonomic assessment including a detailed morphological description and a DNA barcoding analysis. We compared medusa phenotypes from Cochin with those from specimens in its native range and hypothesized that V. gabriellae was introduced to the Kerala coast through hitchhiking on the invasive American Charru mussel Mytella strigata since both species are indigenous to American seas.
Materials and methods
Sample collection
The specimens of limnomedusae referred to as the genus Vallentinia were collected from the laboratory cultures of American Charru Mussel M. strigata used for a salinity and temperature tolerance experiment (Jayachandran et al., Reference Jayachandran, Aneesh, Oliver, Philomina, Jima, Harikrishnan and Nandan2019) in the Department of Marine Biology- Wet lab culture facility. These mussel specimens were originally obtained from Marine Science Boat Jetty-CUSAT, Cochin Estuary 9°96′40.7″N, 76°28′22.6″E 5 October 2019. The salinity and surface water temperature at the collection site was 22 ppt and 30.5°C respectively. The laboratory aquaria contained filtered water encompassing a range of salinities (0, 2, 5, 10, 15, 20, 25, 28, 30, 35, and 40 ppt; Jayachandran et al., Reference Jayachandran, Aneesh, Oliver, Philomina, Jima, Harikrishnan and Nandan2019). During the initial phase of culturing experiment, medusae were not observed. However, after 3 weeks, medusae were observed in tanks with salinities ranging from 20 to 35 ppt and water temperature ranging from 25 to 29°C. Medusae were fed with Artemia nauplii. Five specimens were removed and preserved in absolute ethanol for DNA barcoding. A stereomicroscope was used to monitor the behaviour of live specimens of Vallentinia in seawater. The medusae were then fixed in 5% formalin in seawater and deposited in the Marine Biology Museum of the Department of Marine Biology, Microbiology and Biochemistry, Cochin University of Science and Technology (CUSAT).
Morphological investigations
The living or preserved specimens were subjected to morphological observations and measurements were noted. A stereomicroscope (Magnus MSZ-TR) with an attached digital camera (Nikon D610) was employed to photograph the samples. Detailed morphological measurement was done with a compound microscope (Olympus CX21 and Leica DM6) using standard references (Kramp, Reference Kramp1961; Bouillon et al., Reference Bouillon, Gravili, Gili and Boero2006). Squashes prepared from fresh tissues were examined under a compound microscope to identify nematocysts in medusae (Leica DM6). The following morphological characteristics were documented in this study: umbrella height, umbrella diameter, number of radial canals, exumbrellar tentacles, marginal tentacles, and gonadal structure. Exumbrella tentacles, those growing from the exumbrella at any level, were distinguished from those appearing proximal to the apex – just a short distance from the velum (Goto, Reference Goto1903). Nematocysts were identified according to Ostman (Reference Ostman2000) from collected specimens. Voucher specimens were deposited in the Museum of the Department of Marine Biology, Microbiology, and Biochemistry, School of Marine Sciences, Cochin University of Science and Technology, India (MBM/SBN/HP1/2019).
DNA barcoding
Genomic DNA from ethanol-preserved medusae was extracted using a DNAeasy Blood and Tissue kit (Qiagen) following the manufacturer's instructions. A ~680 bp region of the mitochondrial DNA cytochrome c oxidase subunit I (COI) marker and a ~1 kb region of the 28S rDNA (28S) marker were PCR amplified and sequenced. The PCR mix included 12.5 μl of EmeraldAmp GT PCR Master Mix (Takara Bio), 0.4 μM primers, and 5 ng template DNA in a 25 μl reaction volume. For COI, the degenerate primers dgLCO and dgHCO (Meyer et al., Reference Meyer, Geller and Paulay2005) were used, and for 28S, the primers 28SC1 and 28SC4 (Hassouna et al., Reference Hassouna, Mithot and Bachellerie1984) were used. The amplification conditions for COI involved an initial denaturation step at 95°C for 3 min, followed by 35 cycles of 95°C for 30s, 42°C for 30 s, and 72°C for 1 min, with a final extension of 72°C for 5 min. The conditions were the same for 28S, except for the annealing temperature, which was 52°C. The PCR products were purified by GFX PCR DNA and Gel Band Purification Kit (Cytiva). Bidirectional sequencing was performed with an ABI PRISM Big Dye Terminator v3.1 cycle sequencing kit in an AB 3730 DNA analyser (Life Technologies). The sequences were compiled, aligned, and analysed using Geneious Prime version 2021.0.3 (www.geneious.com). We were only able to obtain sequence data for one medusa, and these were compared with publically available sequences in GenBank using the Standard Nucleotide Basic Local Alignment Search Tool (BLAST). To confirm species identification and visualize genetic distances between closely related species with the COI gene, we downloaded representative olindiid sequences, aligned them using Clustal Omega version 1.2.3, and generated a neighbour-joining tree based on Kimura 2-parameter (K2P) distances using the Geneious PAUP* plugin.
Results
Material examined
Four specimens, sex undetermined, from laboratory culture samples of American Charru Mussel M. strigata collected from Marine Science Boat Jetty-CUSAT, Cochin Estuary 9°96′40.7″N, 76°28′22.6″E collected by Neethu. K.V., 5 October 2019; fixed in 5% formalin.
Identification
The medusae were identified as V. gabriellae Vannucci-Mendes, 1948.
Systematics
Phylum CNIDARIA Verrill, Reference Verrill1865
Class HYDROZOA Owen, Reference Owen1843
Order LIMNOMEDUSAE Kramp, Reference Kramp1938
Family OLINDIIDAE Haeckel, Reference Haeckel1879
Genus Vallentinia Browne, Reference Browne1902
Vallentinia gabriellae Vannucci-Mendes, 1948 (Figure 1)
Morphological description of Indian specimens of V. gabriellae
Medusae are transparent, pale, bell-shaped, and early hemispherical. The umbrella height is about 3.6 ± 0.14 mm and its diameter is about 5.23 ± 0.25 mm (Table 1). Exumbrella is smooth, lacking nematocyst warts (Figure 1A). Four radial canals without branching elongate from four corners of the stomach (Figure 1C), and the ring canal is situated near the umbrellar margin (Figure 1C). No centripetal canals originate from the ring canal. The stomach and manubrium are relatively short with four distinct lips (Figures 1A & E) and the orifice of communication between the stomach and manubrium is relatively broader (Figure 1G) (Table 1). The stomach, with its connecting manubrium, is centrally linked to the radial canals cross-region (Figure 1C). Mouth quadrate to rhomboid and lips deeply folded into four sections (Figure 1E), which continued as shallow ridges on four corners of the manubrium and stomach. There are numerous nematocysts on the mouth rim. The gonads occupy nearly half of the length of the radial canals (Figures 1C & G) and they are clusters of small spherical/papillomaform sacs organized in a zigzag manner (Figure 1H). Two types of tentacles are present – Marginal tentacles and Exumberellar tentacles (Figures 1A & E). Exumberllar tentacles arise from the exumbrellar surface just above the bell margin and are in connection with the ring canal by a hollow endodermal core that passes through the mesoglea (Figure 2A). They are hollow tentacles with scattered nematocysts in their epidermis and provided with suckers and brick red-brown colour at their tips (Figure 1J). There are at least 16–18 such tentacles in our specimen. Marginal tentacles are attached to the subumbrella rim close to the ring canal, and 16–18 tentacles are in each quadrant (Figure 1E). A Large number of nematocyst rings are present in each marginal tentacle and its base (Figure 2C). The oldest of them have an adhesive pad externally near the apex (Figures 1I & J). Only one type of nematocyst is observed, microbasic euryteles (Figure 2D). No ocelli are present; one or two small spherical statocysts are present between each tentacle pair and these statocysts are internal (Figure 2B). Statocysts are hollow closed vesicles and contain a statolith (Figure 2B). Velum has thick muscular layer and an annular ring around its broad central opening (Figure 1E). Velum is always held perpendicular to the main axis. Bell rim flap/lappets are present at the exumbrellar margin of the medusa (Figures 1C & G). Under LED illumination, the endoderm cells at the base of tentacles and bell rim flap/lappets were fluorescent green (Figure 1C). The whole medusa is highly transparent and pale. Ring canal, marginal tentacle, manubrium, and gonads are relatively dark creamy colour in mature medusa (Figures 1A & C). Measurements of V. gabriellae specimens are given in Table 1.
Table 1. Measurements (mm) of live Vallentinia gabriellae

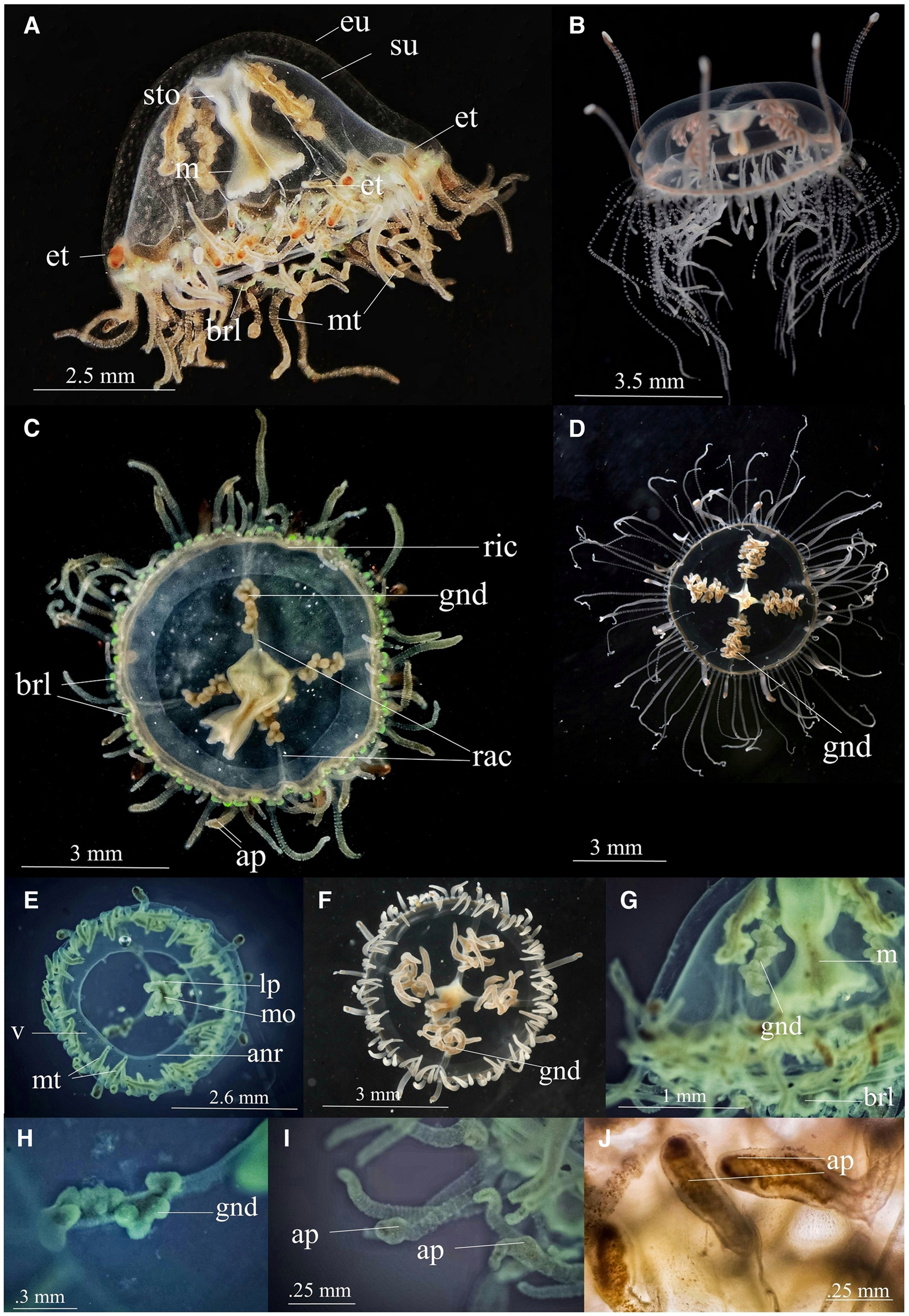
Figure 1. Vallentinia gabriellae (A) lateral view of adult medusa from India (B) lateral view of adult medusa from Florida (C) oral view of adult medusa from India (D) oral view of adult medusa from Florida (E) oral view of juvenile medusa from India (F) oral view of juvenile medusa from Florida (G) lateral view (H) gonad (I) marginal tentacles (J) exumbrellar tentacle. ap, adhesive pad; anr, annular ring; brl, bell-rim lappet; eu, exumbrella; et, exumbrellar tentacle; gnd, gonad; lp, lip; m, manubrium; mo, mouth; mt, marginal tentacle; rac, radial canal; ric, ring canal; sto, stomach; su, subumbrella; v, velum. (Photo courtesy:- B, D & F – Dr Allen G. Collins, Director of National Systematics Lab of NOAA Fisheries and Curator, Smithsonian National Museum of Natural History).
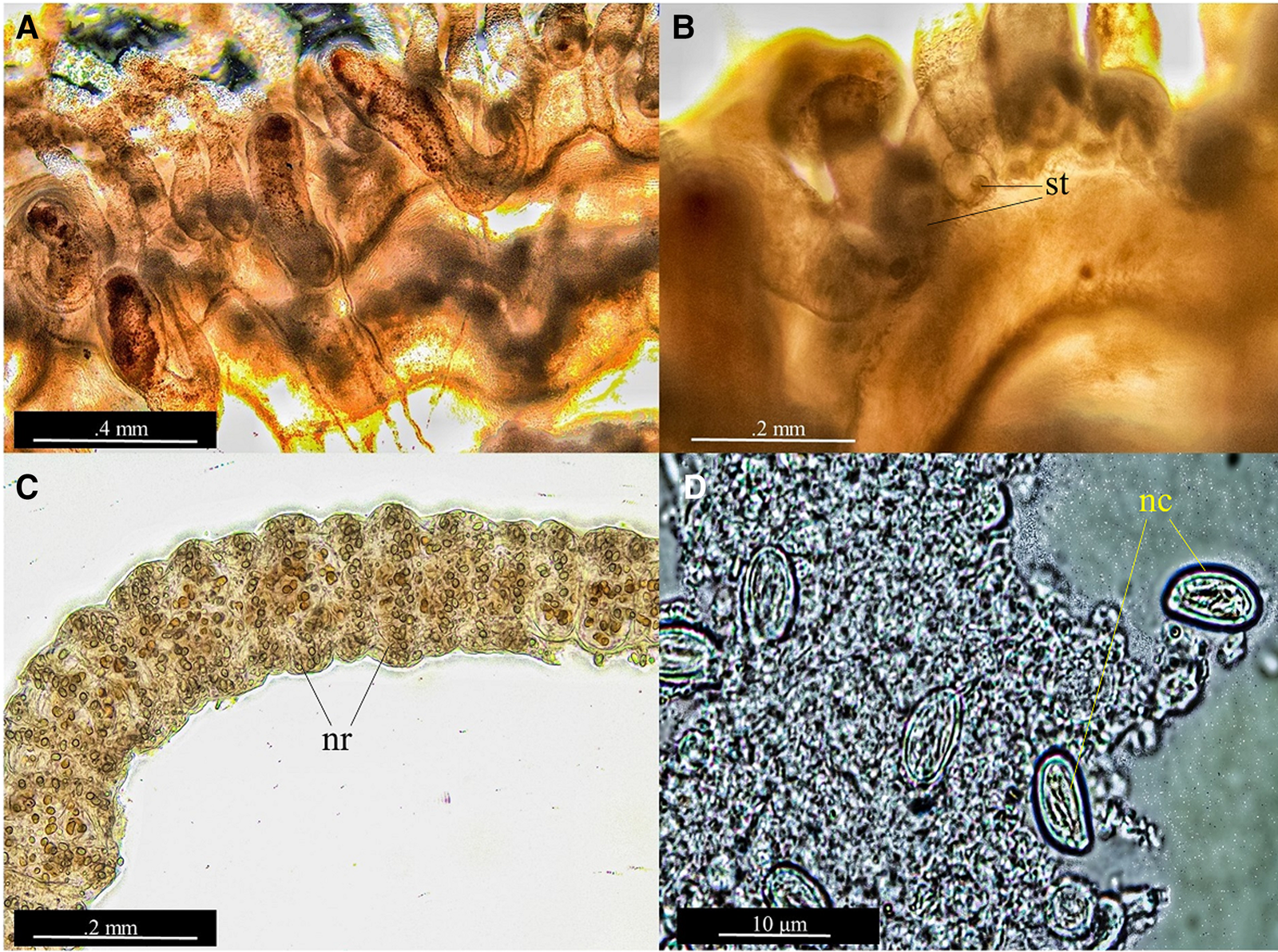
Figure 2. Vallentinia gabriellae, micromorphology of tentacles and nematocysts. (A) exumbrellar tentacle and its endodermal core passes through the mesoglea (B) Statocysts (C) nematocyst ring in the marginal tentacle (D) Microbasic eurytele nematocysts. nc, nematocyst; nr, nematocyst ring; st, statocyst.
DNA barcoding
We generated an 867 base pair fragment of the 28S gene. A BLAST search indicated that this sequence was 100% identical to a V. gabriellae sequence (accession number MG979302; Bentlage et al., Reference Bentlage, Osborn, Lindsay, Hopcroft, Raskoff and Collins2018) for the entire length of our fragment. The GenBank sequence was referred to incorrectly as Cubaia aphrodite in Bentlage et al. (Reference Bentlage, Osborn, Lindsay, Hopcroft, Raskoff and Collins2018) but was subsequently confirmed to be V. gabriellae and the GenBank record was updated (A. G. Collins, pers. comm.). We also generated a 658 base pair fragment of the COI gene. This sequence was >99% identical to a 412 base pair sequence of V. gabriella (411 out of 412 base pairs were identical) on GenBank (accession number MN068283; Matsumoto et al., unpublished). In contrast, it was only ~83% identical to the congeneric V. adherens (accession number OQ589703; A. G. Collins, unpublished), and dissimilar to other published olindiid sequences (Figure 3; Table 2). The K2P distance between V. gabriellae from Kerala and from GenBank was 0.002; while the mean K2P distance between olindiid species belonging to the same genus was 0.155 ± 0.045, and the mean K2P distance between olindiid species belonging to different genera was 0.224 ± 0.073. Both of our sequences were deposited in GenBank (accession numbers: OP800111 for 28S and OP787547 for COI).

Figure 3. Neighbour-joining tree of olindiid species based on Kimura 2-parameter distances using a 412 base pair fragment of the COI gene. Branch lengths are proportional to genetic distances.
Table 2. Pairwise comparisons based on K2P distances between olindiid species included in the study

Habitat and ecology
The original material was obtained from the Cochin estuary, together with the American Charru Mussel M. strigata. The salinity and surface water temperature at the collection site was 22 ppt and 30.5°C, respectively. Medusae are very fond of mussels and they remain attached to their shells (Figure 4) and, on occasion, to the aquaria glass wall with the help of their adhesive pads. The medusa would typically assume a manubrium-up posture, with the adhesive tentacles on the substratum. Individuals would quickly release their hold on the substrate and begin swimming about with the bell's rhythmic pulsations, when prey (Artemia nauplii) was introduced or stimulated. Nematocysts on the filiform marginal tentacles quickly immobilized Artemia. During active swimming, the marginal tentacles contract and move fast with two or three umbrellar strokes, then gently sink to the bottom and cling to the mussel shells. This sedentary epibenthic behaviour could explain how the species has not been previously recorded or even collected using a typical plankton net.

Figure 4. Lateral view of Vallentinia gabriellae medusa attached to shells of Mytella strigata in manubrium-up posture.
Systematic remarks
The exotic variant of V. gabriellae from Indian waters varies from its native forms in the following morphological aspects (Figure 1): V. gabriellae Vannucci-Mendes (1948, p. 73) have reddish-brown, six to twelve-lobed pendant fimbriae like gonad; relatively long and thin and very motile manubrium with four simple lips; orifice of communication between the stomach and the manubrium is very narrow; generally four or eight exumbrellar tentacles with adhesive pad. The exotic form has small spherical/papillomaform clusters of gonads organized in a zigzag manner; relatively short and broad manubrium; broader orifice of communication between the stomach and the manubrium (Table 1); deeply folded four-sectioned lips; at least 16–18 non-perradial exumbrellar tentacles with terminal suckers; bell-shaped medusoid umbrella with bell rim flap/lappets, and fluorescent green at the base of tentacles and bell rim flap/lappets.
Discussion and conclusion
V. gabriellae is a non-indigenous epibenthic hydromedusa that can cling to a wide range of soft and hard substrates in brackish water environments (Mendes, Reference Mendes1948; Foster, Reference Foster1973; Honegger, Reference Honegger1984). There are three accepted species of Vallentinia (Bouillon et al., Reference Bouillon, Gravili, Gili and Boero2006; Schuchert, Reference Schuchert2021): V. falklandica Browne, Reference Browne1902, V. adherens Hyman, Reference Hyman1947, V. gabriellae Vannucci-Mendes, 1948. Except for V. gabriellae, no other species under this genus have been reported from the World Ocean, outside of the original descriptions. V. gabriellae has been reported from mangrove ecosystems on the coast of Brazil, the Caribbean Sea, the Gulf of Mexico, and the Bahamas (Figure 5) (Kramp, Reference Kramp1959; Krumholz, Reference Krumholz1963; Foster, Reference Foster1973; Rey et al., Reference Rey, Kain and De Freese1992; Segura-Puertas and Damas-Romero, Reference Segura-Puertas and Damas-Romero1997; Ramos and Segura-Puertas, Reference Ramos and Segura-Puertas2004; Ahuatzin-Hernández et al., Reference Ahuatzin-Hernández, Canul-Cabrera and Eúan-Canul2020). The euryhaline nature of the group may enable V. gabriellae to survive the wide salinity fluctuations characteristic of impoundment habitats (Rey et al., Reference Rey, Kain and De Freese1992).

Figure 5. Map showing the distribution of Vallentinia gabriellae and Mytella strigata reported so far from the World Ocean.
Our results provide the first documentation of the genus Vallentinia in the Indian Ocean and findings shows that the native and exotic forms of V. gabriellae in this study exhibit certain morphological variations. These include the difference in the gonadal structure, the number of exumbrellar tentacles, the presence of bell rim flaps and lappets, and green fluorescence at its bases, which may be a phenotypic response to the non-native environment. Our identification was enabled by the combination of morpho-taxonomical and molecular approaches. Despite the morphological differences we observed in our specimens and those from its native range, DNA barcoding provided a definitive identification. COI sequences are taxonomically informative at the species level in hydrozoans, exhibiting variability between closely related species while being similar or identical between individuals within a species (Ortman et al., Reference Ortman, Bucklin, Pages and Youngbluth2010). As expected for a species-level match, our sequence was nearly identical to the V. gabriellae sequence on GenBank but relatively distant from all other olindiids, including V. adherens (Table 2).
Other non-indigenous hydrozoans have similarly shown morphological differences in their non-native habitats. Miglietta and Lessios (Reference Miglietta and Lessios2009) reported a swift process of local morphological response similar to this in the hydrozoan T. dohrnii, which can go unnoticed due to rapid morphological change in a new geographic location. Similarly, Da Silveira and Migotto (Reference Da Silveira and Migotto1991) discovered that the hydrozoans Halocardyl disticha (now Pennaria disticha) and Halocardyle fragilis which were previously thought to be two distinct species of Halocardyl, were two extreme morphological varieties of H. disticha due to environmental influences. Phenotypic plasticity may be induced by differences in prey associated with different environments (Caputo et al., Reference Caputo, Fuentes, Woelfl, Castañeda and Cárdenas2021), and future research could assess the role of prey in inducing the morphological variability we observed here.
Hydrozoans may be exceptionally adaptable in terms of surviving transfer and establishing colonies in non-native habitats due to their life history patterns. Transport can occur at any stage of the medusa, polyp, or larval life cycle. We hypothesize that V. gabriellae was transported to Indian waters via polyps on the invasive tropical American brackish water mussel M. strigata. The V. gabriellae polyps have been reported from bivalve shells previously from the Bay of Santos in Brazil (Mendes, Reference Mendes1948). The molluscan shells documented by Mendes (Reference Mendes1948) might include shells of M. strigata because these mussels are common in the estuarine, mangrove, and lagoon environments of Brazil, the Caribbean Sea coast, and the Gulf of Mexico (de Oliveira et al., Reference De Oliveira, Russo, Lazoski, Vianna and Solé-Cava2005) where V. gabriellae has been extensively reported (Kramp, Reference Kramp1959; Krumholz, Reference Krumholz1963; Lytle, Reference Lytle1964; Foster, Reference Foster1973; Rey et al., Reference Rey, Kain and De Freese1992; Ahuatzin-Hernández et al., Reference Ahuatzin-Hernández, Canul-Cabrera and Eúan-Canul2020). This is further strengthened by observations that the habitats of V. gabriellae and Mytella are similar, and that V. gabriellae has to date been reported from locations where Mytella is present or invaded (Figure 5).
Incidental transport of polyps on bivalve shells has been also documented for other non-indigenous hydrozoans. For example, the introduction of Gonionemus sp. into non-native locations may potentially be traced back to the shipment of shellfishes such as Magallana sp., Arcuatula senhousia, and Mytilus galloprovincialis, which carried the benthic stage of Gonionemus (Edwards, Reference Edwards1977; Bakker, Reference Bakker1980; Marchessaux et al., Reference Marchessaux, Gadreaud, Martin-Garin, Thiéry, Ourgaud, Belloni and Thibault2017). Toyokawa and Fujii (Reference Toyokawa and Fujii2015) reported the invasion of two invasive hydromedusae, M. marginata and B. virginica on the coast of Japan, and polyp transport on shells of edible bivalves from China and Korea were proposed as the mechanism of dispersal.
Studies from the Colombian Caribbean (Baptiste et al., Reference Baptiste, Castaño, Cárdenas López, Gutiérrez, Gil and Lasso2010) and the Venezuelan Caribbean (Lodeiros et al., Reference Lodeiros, Hernández-Reyes, Salazar, Rey-Méndez and González-Henríquez2021), as well as reports from the South American coast in the Atlantic from the Gulf of Paria to Argentina and various points in Mexico to Ecuador in the Pacific (Lim et al., Reference Lim, Tay, Lim, Lee, Teo and Tan2018), suggest that M. strigata is a transplanted species in the Caribbean Sea, the Gulf of Mexico, the Florida coast, and the Indian River lagoon. As a basis of our findings, we suspect that the V. gabriellae population reported from this region is introduced, together with M. strigata, and that its native range is limited to the southeast coast of Brazil. M. strigata was recently identified as an aggressive invasive species in Asian nations like the Philippines, Singapore, Thailand, and India (Rice et al., Reference Rice, Rawson, Salinas and Rosario2016; Mediodia et al., Reference Mediodia, De Leon, Anasco and Baylon2017; Vallejo et al., Reference Vallejo, Conejar-Espedido, Manubag, Artiaga, Damatac, Imperial and Cao2017; Lim et al., Reference Lim, Tay, Lim, Lee, Teo and Tan2018; Biju Kumar et al., Reference Biju Kumar, Ravinesh, Oliver, Tan and Sadasivan2019; Jayachandran et al., Reference Jayachandran, Aneesh, Oliver, Philomina, Jima, Harikrishnan and Nandan2019; Sanpanich and Wells, Reference Sanpanich and Wells2019). Given the extensive brackish water systems present on both the east and west coasts of India further records would not be surprising. We suggest that it is probable that V. gabriellae will be introduced to the western Arabian Sea and other sections of the world ocean where M. strigata has been found (Figure 5).
Our results, which establish the presence of V. gabriellae outside Atlantic waters for the first time and document its geographic range expansion, highlight the need of studying hydrozoans with integrative morphological and molecular approaches. We suggest that V. gabriellae could be overlooked in other areas, particularly in non-native locations, due to regional variation in its morphology. We also suggest that future research could include a focus on the polyp stage, and assess its ability to spread to new locations by hitchhiking on the shells of M. strigata and other bivalves.
Data
Data available on request from the authors.
Acknowledgements
The authors are grateful to the Head of the Department of Marine Biology, Microbiology & Biochemistry, Cochin University of Science and Technology for providing the necessary facilities for this study. The authors are thankful to Mr. Aravind E. Haridas for his help during the study and scientific drawings. The authors thank Dr Allen G. Collins (Director of National Systematics Lab of NOAA Fisheries and Curator, Smithsonian National Museum of Natural History) for his help in confirming some morphological characters and sharing pictures of V. gabriellae from Florida. We are also thankful to anonymous reviewers for their suggestions and comments to improve the manuscripts.
Author's contributions
P. H. P., N. K. V., and P. R. J. conducted the fieldwork and collected samples. P. H. P. and N. K. V. prepared the figures and preliminary analysis. P. H. P. and A. F. G. conducted the taxonomic investigation. A. F. G., P. H. P., and A. B. P. conducted the molecular analysis. P. H. P., A. F. G., N. K. V., A. B. P., P. R. J., and S. B. N. analysed the data and wrote the manuscript. All authors participated in revising the manuscript. All authors read and approved the final manuscript.
Financial support
A. F. G. received financial support from the Kathleen M. and Peter E. Naktenis Family Foundation.
Competing interest
None.
Ethical standards and consent to participate
Not applicable.