INTRODUCTION
Reliable radiocarbon dating results require knowledge about the origin and distribution of 14C in different environmental compartments. In an aquatic environment, the dissolution of carbon dioxide through the air/ocean interface is responsible for the presence of radiocarbon in the oceans. This process is not uniform across the oceans, and in addition, marine currents also contribute to variations in 14C activities at different water samples. Surface waters have 14C higher activities than deep waters (Alves et al. Reference Alves, Macario, Souza, Pimenta, Douka, Oliveira, Chanca and Angulo2015a, Reference Alves, Macario, Ascough and Bronk Ramsey2018; Sikes et al. Reference Sikes, Cook and Guilderson2016).
Because of these factors, it is not possible to directly compare the radiocarbon ages of contemporary terrestrial and marine samples. Marine samples present older radiocarbon ages caused by the uptake of 14C that has already suffered radioactive decay due to long residence times in deep oceans, comprising the so-called “marine reservoir age R (t)” (Evin et al. Reference Evin, Marechal, Pachiaudi and Puissegur1980; Goodfriend and Stipp Reference Goodfriend and Stipp1983; Goslar and Pazdur Reference Goslar and Pazdur1985; Yates Reference Yates1986). This scenario is even more complex in estuarine environments due to the influence of freshwater intake and ocean dynamics (Goodfriend and Flessa Reference Goodfriend and Flessa1997; Ulm Reference Ulm2002). Thus, whenever 14C measurements are performed on sea-influenced material, a correction is necessary to compare with terrestrial samples, but because of complexities in ocean circulation the actual correction varies with location. This regional difference from the average global marine reservoir correction is designated ΔR (Stuiver and Braziunas Reference Stuiver and Braziunas1993).
The reservoir effect (ΔR) parameter measures the variation in marine reservoir age between regions. It is the difference of local marine reservoir age in relation to the globally averaged mixed-layer reservoir age (Rglobal), which exhibits a mean value of around (500±60) during the last 11.6 kyr (Fischer and Olsen Reference Fischer and Olsen2021). Besides being an important correction for marine sample 14C ages (Stuiver et al. Reference Stuiver, Reimer and Braziunas1998), the ΔR can also be employed as an upwelling effect indicator. High positive values can be correlated to strong upwelling events, whereas low or negative ΔR values correspond to weak or non-existent upwelling events (Diffenbaugh et al. Reference Diffenbaugh, Sloan and Snyder2003). But the upwelling is not the only reason for these differences, we need take into account species-specific effects, habitat and/or substrate too, which can impact local ΔR determinations (Lindauer et al. Reference Lindauer, Santos, Steinhof, Yousif, Phillips, Jasim, Uerpmann and Hinderer2017; Petchey Reference Petchey2020).
Coastal locations are highly influenced by hydrographic conditions, environmental factors and sources of terrestrial carbon which can be expected to modify the local reservoir age R(t) (Lougheed et al. Reference Lougheed, Filipsson and Snowball2013). Numerous research around the world, for example, in Atlantic Iberia (Soares Reference Soares and Martins2010), the Baltic Sea (Lougheed et al. Reference Lougheed, Filipsson and Snowball2013), and southern and eastern South Africa (Maboya et al. Reference Maboya, Meadows, Reimer, Backeberg and Haberzettl2018) indicates the variability of ΔR in coastal waters.
In freshwater rivers and lakes, there is a similar effect to the marine reservoir effect on radiocarbon dates. It is known as the freshwater reservoir effect (FRE). In these systems, carbon comes from two sources: CO2 from the atmosphere and dissolved inorganic carbon (DIC) from the groundwater (Wood et al. Reference Wood, Higham, Buzilhova, Suvorov, Heinemeier and Olsen2013), being those arising from the dissolution of carbonate minerals is the largest source of “old” carbon in freshwater (Svyatko et al. Reference Svyatko, Mertz and Reimer2015).
One of the ways to determine the marine reservoir age R(t) is by dating paired marine and terrestrial samples from contemporary archaeological contexts (Head et al. Reference Head, Jones and Allen1983; Dettman et al. Reference Dettman, Mitchell, Huckleberry and Foster2015; Hadden and Cherkinsky Reference Hadden and Cherkinsky2015; Latorre et al. Reference Latorre, De Pol-Holz, Carter and Santoro2017). The local reservoir age R(t) is determined by the difference between these ages, and it is expressed for a specific location and calendar age t. The regional corrections of the R(t) are reported as ΔR values, i.e.,

Once calculated, ΔR can be applied to to the marine calibration curve (Marine20, Heaton et al. Reference Heaton, Köhler, Butzin, Bard, Reimer, Austin, Ramsey, Grootes, Hughen, Kromer and Reimer2020) to calibration of marine 14C dates. Despite its huge extension, few studies concerning the marine reservoir effect for the Brazilian coast are available (Alves et al. Reference Alves, Macario, Souza, Pimenta, Douka, Oliveira, Chanca and Angulo2015a, Reference Alves, Macario, Souza, Aguilera, Goulart, Scheel-Ybert, Bachelet, Carvalho, Oliveira and Douka2015b; Carvalho et al. Reference Carvalho, Macario, De Oliveira, Oliveira, Chanca, Alves, Souza, Aguilera and Douka2015; Macario et al. Reference Macario, Souza, Aguilera, Carvalho, Oliveira, Alves, Chanca, Silva, Douka, Decco and Trindade2015, Reference Macario, Alves, Chanca, Oliveira, Carvalho, Souza, Aguilera, Tenório, Rapagnã, Douka and Silva2016).
Shell mounds are the most recognized and studied archeological hill-shaped sites in Brazil, and the object of intense research by Brazilian archeology (Gaspar et al. Reference Gaspar, Klokler and De Blasis2011, Reference Gaspar, Klokler and Bianchini2013, Reference Gaspar, Klokler and DeBlasis2014; Klokler Reference Klokler2014; Klokler et al. Reference Klokler, Gaspar and Scheel-Ybert2018). These mounds were built by fishers and collectors who settled throughout coastal, estuarine and riverine areas, in islands, lagoons, coves, peninsulas, salt marshes, as well as some river courses. They comprise small or large elevations, which in some regions may reach over 70 m high and 500 m long, defined as often stratified deposits of shells, fish bones, lithic and bone artifacts and charcoal, which have been interpreted as funerary spaces (Bianchini et al. Reference Bianchini, Gaspar, De Blasis and Scheel-Ybert2011), due to the huge concentrations of human burials and the absence of domestic areas (Gaspar Reference Gaspar1991; Barbosa Reference Barbosa2007). In the last decade, research carried out in the states of São Paulo and Rio de Janeiro has confirmed the function of these sites as cemeteries, following the identification of funeral areas, where burial concentrations are located (Gaspar et al. Reference Gaspar, Klokler and Bianchini2013).
In the present study, R(t) values obtained from paired shell (marine) and charcoal fragment (terrestrial) samples from the Sernambetiba and Amourins shell mounds at Guanabara Bay are reported (Figure 1). Radiocarbon ages were obtained from marine samples from the Guapi site, located near the Amourins site (Figure 1). ΔR value was determined by subtracting the local reservoir age R(t) value from the globally average mixed layer reservoir age Rglobal. Based on the obtained ΔR results, calibrated 14C ages were calculated for the two investigated shell mound sites. The research area is located in the northern portion of the wide sedimentary plain of Guanabara Bay and covers about 7000 km2. In this location, twenty shell mound sites are known and registered by the University of Rio de Janeiro National Museum (MN/UFRJ).

Figure 1 Map displaying the location of the Sernambetiba, Amourins, and Guapi shell mounds near Guanabara Bay.
According to Amador (Reference Amador1997), the surrounding Guanabara Bay region attracted human occupation, due to the availability of a wide variety of marine foods, such as fish, crustaceans, mollusks, and seaweed, among others, as well as terrestrial foods such as fruits, mammals and reptiles. In addition, freshwater availability from rivers that flow into the Guanabara Bay and forests used to obtain wood material is also noteworthy.
As explained by Amador (Reference Amador1997), around 18,000 cal BP, during the last glacial maximum, the sea level was about 130 m below its current level, maintaining the local coastline tens of kilometers outward compared to the current margins. Guanabara Bay displayed river features during this period, comprising the Paleo-Rio-Guanabara. From 18,000 cal BP, due to gradual global warming and progressive melting of the polar ice caps at high latitudes, several transgressive events took place, partially interrupted by minor regressions and coastline stabilization, followed, a rapid rise in sea level. At about 7948–7598 cal BP, the sea level was 0.5 m below its current level, which was first reached in the Holocene around 7500 cal BP. The maximum level of 3.0 m above the current level was reached between 4787 and 4104 cal BP (Castro et al. Reference Castro, Seoane, Fernandes, Cabral, da Cunha, Malta, Miguel, de Oliveira, de Oliveira and de Souza Tamega2021). The sea then advanced, reaching the base of the Serra do Mar Mountain Range and areas which are now located 30 km distant from the coast. At around 4500 cal BP, the sea level began its retreat, until reaching the current level.
At that time, restingas and lagoons were present, local mangroves expanded and dunes, marshes and salt marshes were formed, progressively reducing the surface of the bay until reaching modern settings (Castro et al. Reference Castro, Suguio, Cunha and Dias2014). Mangroves exhibited rapid expansion, mainly in the Macao, Guapi-Açu and Guaxindiba river basins, all surrounding Guabanara Bay, and in the basins of other rivers that flow into the area (Amador Reference Amador1997).
Several shell mound records are noted for the surrounding Guanabara Bay region. About 20 are situated in the Northeastern portion of this area, indicating that the region was densely occupied by ancient groups. Due to the small average distance between settlements (approximately 6 km) and some concomitant dates available in the literature, routine contact between groups can be inferred (Souza et al. Reference Souza, Liryo, Bianchini and Gaspar2012; Gaspar et al. Reference Gaspar, Klokler and Bianchini2013; Bianchini Reference Bianchini2015; Klokler et al. Reference Klokler, Gaspar and Scheel-Ybert2018).
MATERIAL AND METHODS
Samples
Samples from the Sernambetiba (16 mollusk shells, Anomalocardia brasiliana and Lucina pectinata, and 3 charcoal fragments), Amourins (7 mollusk shells, Lucina pectinate, and 3 charcoal fragments), and Guapi (20 Ostrea shells) shell mounds were investigated (Figure 1). All samples were obtained from the Federal University of Rio de Janeiro National Museum (MN/UFRJ) archaeological collection. Due to the fire that destroyed the National Museum on 9/2/2018, some of these samples became unique, in particular, those from the Amourins shell mound, whose archaeological site is not well preserved (Gaspar et al. Reference Gaspar, Klokler and Bianchini2013). Figure 2 presents the study region located on the southeastern Brazilian coast and other regions studied by different authors.
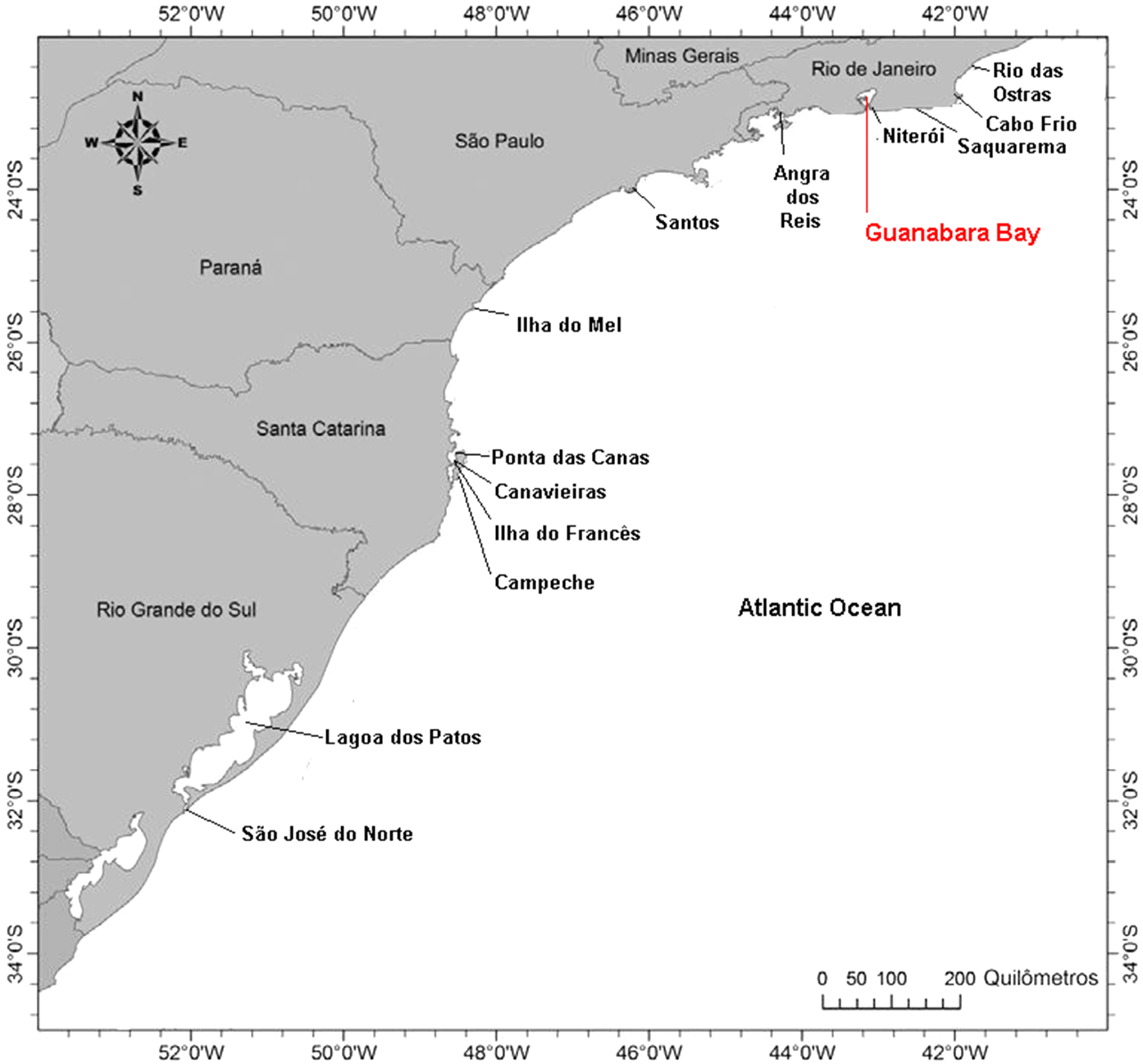
Figure 2 Study region located on the southeastern Brazilian coast (red) and regions studied by other authors (black). (Please see online version for color figure.)
The Amourins site was reviewed by Gaspar et al. (Reference Gaspar, Klokler and Bianchini2013) and has an age of about 4000 years. Five graves have been identified at Amourins and how they were built was described by Berredo et al. (Reference Berredo, Gaspar, Ramos and Bianchini2020). To seal the graves, there is a sequence of three layers built from ashes, charcoal pieces, fish bones and shells, mainly, Lucina (Lucina pectinate). Four Lucina pectiana shells and charcoal samples came from the seal of the graves identified at the profile 30–35, at a depth of about 1.30 m (Gaspar et al. Reference Gaspar, Klokler, Sheel-Ybert and Bianchini2013).
The Sernambetiba site is much younger than Amourins, circa 2000 years (Bianchini Reference Bianchini2015). It is composed, in fact, by several sub-hills, each one containing several graves. According to this author, at the Sernambetiba site there is three excavation areas, named locus, and the actual samples came from locus 2 and 3. As a result of how this sambaqui was built and operated, there is not a large time difference according to the sample stratigraphy. Bianchini (Reference Bianchini2015) has observed charcoal samples with the same age (circa 1900 years) at the surface (0.30 m) and at 5.00 m depth. Anomalocardia brasiliana is the predominant specie but lucinas are present on the top layer that covers the structure.
The Guapi site is the oldest of these three (5000 years) and less studied than Amourins and Sernambetiba, the observed references are internal Nacional Museum report and a M.Sc. dissertation (Pinto Reference Pinto2009; Borges Reference Borges2015).
Radiocarbon Dating
All samples were treated according to Pessenda and Camargo (Reference Pessenda and Camargo1991) and dated at the 14C Laboratory, located at the Radioprotection and Dosimetry Institute, belonging to the Brazilian National Nuclear Energy Commission (IRD/CNEN). Dating was carried out via the benzene synthesis methodology and 14C activities were determined by liquid scintillation spectrometry. International Atomic Energy Agency carbonate reference standards (IAEA C-2; pMC = 23.05; δ13C = –25.5‰). wood (IAEA C-5; pMC = 41.14; δ13C = –8.3‰), and marble samples were also submitted to the same procedure (Pessenda and Camargo Reference Pessenda and Camargo1991; Beramendi-Orosco et al. Reference Beramendi-Orosco, Gonzalez-Hernandez, Urrutia-Fucugauchi and Morton-Bermea2006; Bronić et al. Reference Bronić, Horvatinčić, Barešić and Obelić2009; Baydoun et al. Reference Baydoun, Samad, Aoun, Nsouli and Younes2014).
The samples (40 g of shell and 10 g of charcoal) were pretreated to eliminate potential interferences and to separate the carbon phase of interest. Contamination may originate from sample preparation steps, such as collection and handling, adhering to the samples, or through carbon exchanges. Complete shells were physically pretreated by scraping to remove adhered materials using spatulas, brushes, and tweezers, and chemically by immersion in 4% hydrochloric acid followed by three successive washings with deionized water. Charcoal fragments were treated by the acid-alkaline-acid (AAA) method. This method consisted of treating samples with 1 mol L–1 hydrochloric acid (HCl) at 50°C for 4 hr, 0.1 mol L–1 sodium hydroxide (NaOH) at 50°C for 4 hr to remove soluble humic acids, and 1 mol L–1 HCl at 50ºC for 4 hr. Samples were neutralized by washing with distilled water after each acid and alkali wash (Pessenda and Camargo Reference Pessenda and Camargo1991). All the samples were dried at 60ºC for 24 hr and ground.
The benzene synthesis system concentrates 92% of the carbon present in samples into this organic molecule. The conversion, which takes place in a closed system under vacuum (TASK benzene synthetizer, Athens, Georgia, USA) initially converts all sample carbon into carbon dioxide. In the case of shell samples, gas formation occurs through the slow addition of 1:1 phosphoric acid, while gas formation occurs through the combustion in a closed system (Parr pump) pressurized with oxygen to a pressure of 200 psi for the charcoal fragments samples. Subsequently, lithium carbide is formed by reacting the carbon dioxide with molten lithium at 850°C and acetylene is formed by reacting lithium carbide with water. In the last step, the acetylene is trimerized by catalysis with vanadium pentoxide, producing benzene.
The synthesized benzene was immediately transferred to a 7 mL vial and mixed with a 0.5 mL scintillation solution containing 43.75 g PPO (2,5-diphenyloxazole) and 2.59 g POPOP [1-4,bis-2-(5-Pheniloxazolyl)-benzene] for measurement. The samples and standards were measured for 24 hours applying a low-level liquid Perkin-Elmer Quantalus 1220 scintillation counter. The counting efficiency for 100 pMC, based on both IAEA 14C certified reference materials, was 10.72 (0.18) counts per gram carbon and the background was 1.0 count per minute. The δ13C values were determined by IRMS at the Stable Isotopes Laboratory, in the University of Brasília Geosciences Institute.
Ages were calculated according to Stuiver and Polach (Reference Stuiver and Polach1977) and correspond to conventional radiocarbon ages. Marine reservoir age R(t) was calculated by the difference between the radiocarbon ages of paired marine and terrestrial samples nearby and in the same layer from the archaeological site. ΔR value was determined by subtracting the local reservoir age R(t) value from the globally average mixed layer reservoir age Rglobal.
The radiocarbon ages uncertainties (k=2) comprise expanded propagated uncertainties, including reproducibility, calibration, sample, and blank counting uncertainties. Ages calibration was performed using the OxCal version 4.4 software (Bronk Ramsey Reference Bronk Ramsey2009). Calibrated ages of the marine samples were obtained using Marine20 curve (Heaton et al. Reference Heaton, Köhler, Butzin, Bard, Reimer, Austin, Ramsey, Grootes, Hughen, Kromer and Reimer2020) with application of a local reservoir adjustment, while SHCal20 curve was used for the charcoal samples (Hogg et al. Reference Hogg, Heaton, Hua, Palmer, Turney and Southon2020). The applied equations for the radiocarbon ages and the reservoir effect, together with the uncertainty calculation, are presented as Supplementary Material.
RESULTS AND DISCUSSION
Radiocarbon Ages and the Reservoir Effect
The obtained radiocarbon and calibrated ages for the Sermambetiba and Amourins mounds are presented in Tables 1–2. Radiocarbon ages for Guapi shell mound are presented in Table 3.
Table 1 Sernambetiba shell mound: shell and charcoal sample radiocarbon and calibrated ages and their respective δ13C values.

* For Anomalocardia.
Table 2 Amourins shell mound: shell and charcoal sample radiocarbon and calibrated ages and their respective δ13C values.

Table 3 Guapi shell mound: shell sample radiocarbon ages and their respective δ13C values.

Samples from the Sernambetiba site presented a very narrow age range for charcoal fragments (2480–2280 14C BP). The data reveal a mean value of 2586 14C BP and an error of the mean of 40 years for Anomalocardia shells, and a mean value of 2357 14C BP and an error of the mean of 60 14C yr for charcoal fragments, resulting in a marine reservoir age of 229 ± 72 14C yr. The marine reservoir age was also calculated pairing shell and charcoal fragment samples, obtaining a value of 256 ± 37 14C yr, although both values were statically equal.
On the other hand, shell samples from the Amourins site presented a broader age range (4610–3560 14C BP), while charcoal samples presented a narrower age range (4080–3850 14C BP). Four Lucina pectnata shells located near the charcoal samples were used to calculate the marine reservoir age. A mean age value of 4382 ± 100 14C BP and a mean of 3970 ± 70 14C BP were obtained to marine and terrestrial samples, respectively. These results provide a marine reservoir age of 412 ± 118 14C yr. The obtained reservoir age based on shell and charcoal fragment pairing was of 413 ± 67 14C yr, with both values statically equal.
Comparing the ages of both sites, Amourins corresponds to a period of higher sea level compared to Sernambetiba (Amador Reference Amador1997) and, therefore, subjected to a lesser estuarine influence. Furthermore, a higher sea level denotes a larger reservoir effect, as observed herein. Applying (500 ± 60) yr as RGlobal Av, for the last 11.6 kyr (Fischer and Olsen Reference Fischer and Olsen2021), the calculated ΔR values were –87 ± 90 and –244 ± 70 14C yr for Amourins and Sernambetiba shell samples, which were used to calibrate marine sample ages for the investigated archaeological sites. Table 4 compared the obtained ΔR values to those reported in the literature. All values were calculated based on the MARINE20 curve (Heaton et al. Reference Heaton, Köhler, Butzin, Bard, Reimer, Austin, Ramsey, Grootes, Hughen, Kromer and Reimer2020). The values observed herein are consistent with published values for the Rio de Janeiro state coastal region (Alves et al. Reference Alves, Macario, Souza, Aguilera, Goulart, Scheel-Ybert, Bachelet, Carvalho, Oliveira and Douka2015b; Carvalho et al. Reference Carvalho, Macario, De Oliveira, Oliveira, Chanca, Alves, Souza, Aguilera and Douka2015; Macario et al. Reference Macario, Souza, Aguilera, Carvalho, Oliveira, Alves, Chanca, Silva, Douka, Decco and Trindade2015; Macario et al. Reference Macario, Alves, Chanca, Oliveira, Carvalho, Souza, Aguilera, Tenório, Rapagnã, Douka and Silva2016).
Table 4 Comparison between published marine reservoir effects along the Rio de Janeiro state coast and the present assessment.*

* The values herein from the literature are the adjusted values from calib.org/marine due to the new global marine R.
The Guapi shell mound represents a long-lasting site, with radiocarbon ages ranging from 4590–5490 14C BP. As pointed out by Gaspar et al. (Reference Gaspar, Klokler, Sheel-Ybert and Bianchini2013) the existence of shell mound sites displaying millennial occupation history reinforce the hypotheses that the shell mound peoples achieved a very large population, supporting the growth of the shell mound itself but also their cultural values. Comparing the shell mound site location (Figure 1) to shell ages, the distance to the actual coastal line follows an age sequence, where the older site (Guapi) is located farther to the actual coastal line than the younger site (Sernambetiba), corroborating seawater level variations throughout the last 12,000 years (Castro et al. Reference Castro, Suguio, Cunha and Dias2014). Amourins, displaying an intermediate age, is located closer to Guapi than to Sernambetiba, verified by their age difference.
Figure 3 presents the age distribution for the investigated Guanabara Bay shell mounds, including values of this study as well as existing literature data. A continuous existence of shell mound peoples is, thus, verified around Guanabara Bay, lasting, at least, 4000 years, beginning about 6000 years ago and ending circa 1800 years ago when the arrival of more belligerent populations, such as the Tupi-Guarani, led to the end of their civilization (Macario et al. Reference Macario, Souza, Trindade, Decco, Lima, Aguilera, Marques, Alves, Oliveira and Chanca2014). Although they continuously inhabited this region, as it is a very flat area, these peoples were affected by sea-level changes, moving periodically to avoid long distances to the shoreline.

Figure 3 This study and published Guanabara Bay radiocarbon shell mound ages.
CONCLUSIONS
This study aimed to expand knowledge concerning the poorly studied marine reservoir effect and paleoclimatic conditions along the western South Atlantic coast, specifically at Guanabara Bay. ΔR values were obtained employing paired shell mound samples from two archaeological sites in this region during the middle to late Holocene. The marine reservoir effects for this estuarine system were ΔR = –87 ± 90 14C yr and ΔR = –244 ± 70 14C yr for 3970 ± 70 yr BP and 2357 ± 60 yr BP, respectively, probably due to the increased influence of fresh water over the period. These new results are coherent with previously published values for the Rio de Janeiro state coastal region.
Samples from the two archaeological sites at Guapimirim (Sernambetiba and Amourins) Rio de Janeiro, were dated using the obtained ΔR values, and from one site (Guapi) radiocarbon ages were presented, contributing with the age database concerning shell mounds at Guanabara Bay Region.
ACKNOWLEDGMENTS
The authors would like to thank Maria Dulce Gaspar and Gina F. Bianchini (Museu Nacional/Universidade Federal do Rio de Janeiro), who kindly provided the shell mound samples.
Supplementary material
To view supplementary material for this article, please visit https://doi.org/10.1017/RDC.2022.73