BC43 Benchtop Confocal System
Andor Technology, an Oxford Instruments company
Developers: Allister Pattison, Stephen McKenzie, David Cooper, Darren Frew, Aidan Harkin, Arash Vakily, Conor Hamill, David Gould, Paul Armstrong, Aaron McCulloch, and Gerry Collins
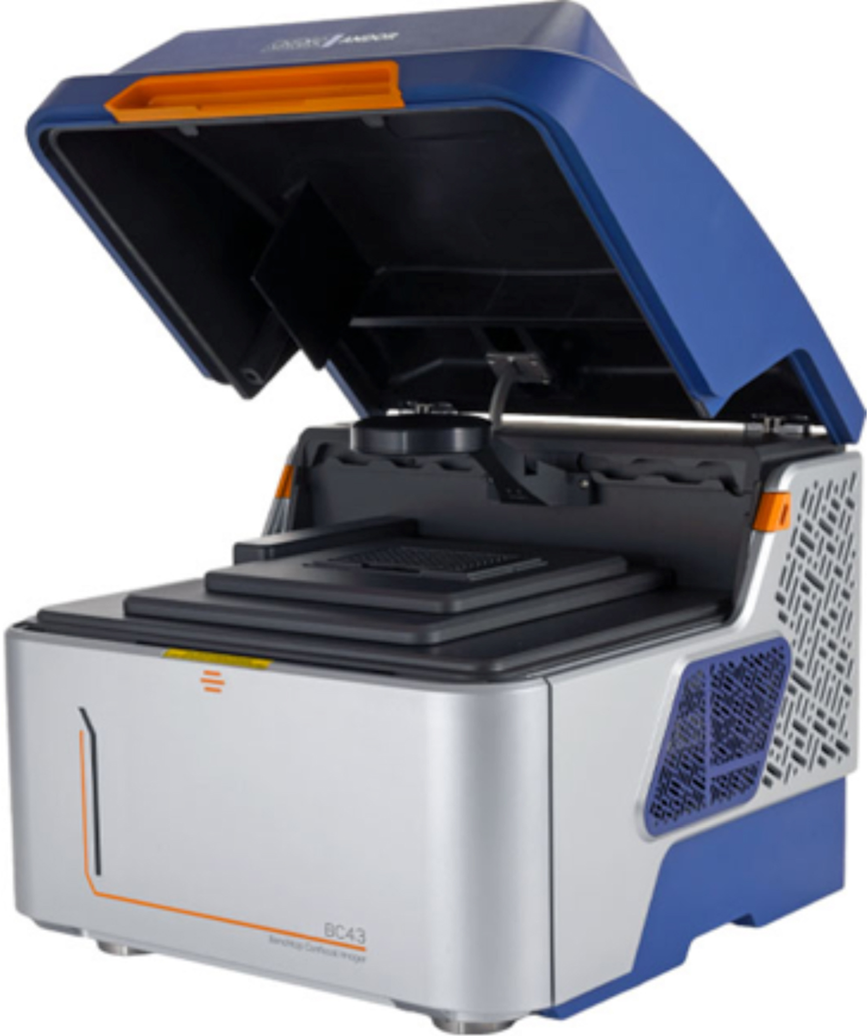
The BC43 is a high-performance benchtop light microscope that does not require a dark room or microscopy expertise for its operation. The BC43 provides high-speed Nipkow disk confocal microscopy in a benchtop instrument with a small footprint. It also incorporates patented Focus Seek and Lock technology that can auto-find the surface on which a sample is mounted and apply an offset to a focal point just within the sample. The same software can then lock to that surface to maintain focus during time-lapse experiments and multi-field imaging. The instrument integrates three imaging modes: (1) high-speed confocal using micro-lens-based spinning disk technology with a high-efficiency sCMOS sensor to allow fast scanning and short exposures. A combination of special disk design and patented Borealis illumination optimizes tiled imaging of large and thick samples up to several hundred micrometers deep; (2) widefield epifluorescence can be used when a sample is particularly sensitive to light, when the highest frame rates are needed, or if the confocal mode limits signal from a weakly labeled sample; (3) brightfield either using simple transmitted light, which is often of limited use unless the sample has its own structural contrast, or Andor's Differential Phase Contrast (DPC), which obliquely illuminates the sample from multiple angles, creating the appearance of three-dimensional physical relief. DPC does not rely on polarized light, allowing use of plastic vessels.
By integrating a high-speed, high-performance microscope with three key imaging modalities into a cost-effective benchtop instrument, researchers are now able to include confocal, and therefore three-dimensional imaging, as part of their routine workflow in the same laboratory space as sample preparation and other activities. Ease-of-use with a short learning curve means that students and post-doctoral researchers alike can benefit from both improvements in data quality and productivity since the system images about 10 times faster than conventional point scanning confocal, at a like-for-like resolution and field-of-view.
Key applications will be microscopy of large samples, including 3D cell cultures and whole model organisms, such as zebrafish and Drosophila, as well as imaging in multicellular environments typical of disease states encountered in cancer, kidney, and brain research.
X-ray Perimeter Array Detector
Argonne National Laboratory and Thermo Fisher Scientific Instruments
Developers: Nestor Zaluzec, Mikhail Ovsyanko, and the Eindhoven UltraX Development Team

The X-ray Perimeter Array Detector (XPAD) is an advanced silicon drift detector (SDD) for detecting specimen characteristic X-rays in the transmission electron microscope and is marketed under the name Ultra-X. The challenge is to detect the most X-rays from a tiny region of a thin specimen given the limited physical space at the objective lens of a modern aberration-corrected TEM or STEM. The number of specimen X-ray counts per nanoampere (nA) per second is a key figure-of-merit. The silicon drift detector (SDD) has several advantages related to the challenge: it counts X-rays quickly, it does not require liquid nitrogen cooling, and the counts from multiple detectors can be combined. The XPAD makes use of all these aspects in a proprietary configuration that yields an effective X-ray collection angle of > 4 steradians (sR). Thus, this new detector provides a 600% improvement in X-ray collection over the typical single SDD (~ 0.6 sR) designed for this purpose.
With the X-ray collection solid angle, and hence signal, increased to the maximum possible, two notable benefits are realized. First, for the same experimental conditions, the statistical quality of any measurement is correspondingly increased, or alternatively, the acquisition time is reduced at constant statistics. This simple advance improves the quality of X-ray data at all levels, as well as the ability to acquire hyperspectral compositional images in time scales as short as a few hundred μsec/pixel with beam currents of a few tens of picoamperes (pA). Secondly, the minimum detectable mass fraction (MMF) of an element improves (decreases) at higher X-ray peak intensity (P) for that element. Since P increases with collection angle, smaller amounts of an element can be detected compared with previous detectors.
Innovations in microanalysis should provide results that are quicker, better, or cheaper. Data that once took a minute to acquire can now be obtained in under 10 seconds. Compared with previous detectors under similar electron beam conditions, data can be collected with six times better statistics. Finally, while the new detector may cost more, if operator time is considered, quality results can be obtained more quickly, yielding cost savings over the long term.
Multilayer Laue Lens Optic for Hard X-ray Microscopy
Brookhaven National Laboratory and the Naval Research Laboratory
Developers: Wei Xu, Weihe Xu, Nathalie Bouet, Juan Zhou, Hanfei Yan, Xiaojing Huang, Ming Lu, Maxim Zalalutdinov, Yong Chu, and Evgeny Nazaretski

This X-ray microscopy project involves a novel two-dimensional nano-focusing device that combines linear multilayer Laue lenses (MLLs) with MEMS (micro electro-mechanical systems) technology, yielding a compact way to achieve nano-scale imaging resolution in the hard X-ray regime. Multilayer Laue lenses are fabricated as one-dimensional focusing elements. A pair of two independent lenses is required to perform point-focusing. In order to achieve an astigmatism-free point focus of ~10 nm and below, two lenses have to be aligned orthogonally with respect to each other better than 10 millidegrees. In addition, the spatial placement of individual lenses along the X-ray beam must be better than the depth of focus (typically ~5 microns or less above 12 keV photon energy). At present, extremely complex and sophisticated alignment devices have been designed and constructed to satisfy these requirements. The developed 2D MLL/MEMS-based device overcomes the alignment complexity within an X-ray microscope. The MEMS-based optic is nearly immune to thermal drifts, and it enables a much more compact X-ray microscope design with higher resonance frequencies, leading to cost savings on vibration isolation infrastructure.
By leveraging the high accuracy of microfabrication technology, the orthogonality requirement between two MLLs has been satisfied to 5 millidegrees, and the lateral position along the X-ray beam direction has been controlled to micrometer levels. This optic produced a point focus of ~13 nm at the Hard X-ray Nanoprobe Beamline of NSLS-II. As of today, few attempts to pre-package two independent 1D MLLs and achieve diffraction-limited focus have been successful; the work here constitutes the first demonstration of a user-friendly 2D nano-focusing device for hard X-rays.
Any third-generation synchrotron facility that operates a hard X-ray zone plate-based microscope could benefit from these new focusing devices. Dozens of synchrotron-based microscopes around the globe could be equipped with 2D MLL optics. That, in turn, would improve hard X-ray imaging capabilities for materials, energy, and bioscience studies. Moreover, the MLL optic described here could save costs associated with conventional MLL-based microscopes that require elaborate vibration-isolation infrastructure. This 2D MEMS-based MLL optic is a significant step forward, bringing robust, compact, user-friendly, high-resolution hard X-ray imaging capabilities to synchrotron- and lab-based X-ray microscopes.
Invizo 6000® Wide-Field Atom Probe
CAMECA Instruments Inc.
Developers: Dan Lenz, Joe Bunton, David Larson, and David Reinhard

The Invizo 6000® is an improved three-dimensional atom probe tomography (APT) system, a sensitive analytical microscope for nanoscale 3D analysis of materials. In all atom probes, a sharply pointed specimen is kept at a high positive potential in an ultra-high vacuum system. The specimen is also kept at cryogenic temperatures to stop surface diffusion. Individual ions are liberated from the surface and drawn to the detector with either an electric pulse or a laser pulse; the latter raises the specimen temperature in order to increase ionization probability. This new analytical instrument introduces several breakthrough technologies, including a patented electrostatic ion flight path design that simultaneously enables an increased field-of-view (FOV) and enhanced mass resolving power, a deep UV (DUV) laser to promote higher yield and data quality, and advanced dual-beam beam delivery optics to improve specimen symmetry and reconstruction fidelity. These features provide larger analysis volumes, enhanced specimen yield, and improved sensitivity—all of which expand analytical capabilities. For the user this means easier specimen preparation, increased likelihood of successful analyses, and nearly four times more data (wider FOV) with no loss in spectral quality. These advances, particularly the wider FOV, provide better results more quickly.
Practitioners of atom probe tomography have historically been limited by a small field-of-view showing only fractions of microscale features, low counting statistics, a slow time-to-knowledge, and either a large field-of-view (FOV) or a high mass resolving power—but not both. These hurdles limited the adoption of atom probe tomography despite the power of the data. The Invizo does not just address one or two of these limitations but addresses all of them simultaneously.
Like all atom probe tomography systems, the Invizo 6000® will be used on a wide variety of metals, semiconductors, and insulators, including materials with complex phases like superalloys and functional materials like those in modern microelectronic devices. The larger FOV (hundreds of nanometers in diameter), approaching that of the TEM, allows more accurate correlation to materials properties obtained by modeling and simulation. The Invizo will not only open new applications, but also will solve more difficult problems.
DeepRecon Pro Deep-Learning Software for X-ray Microscopy
Carl Zeiss Microscopy
Developers: Andriy Andreyev, Matthew Andrew, Faguo Yang, Lars Omlor, Christoph Graf Von Hagen, Ravikumar Sanapala, Robin White, Ozair Rajani, and Sam Kalirai

DeepRecon Pro is a novel tomographic image reconstruction algorithm for microCT and X-ray microscopy that yields image quality improvements and noise reduction at reduced acquisition times. By combining a tomographic reconstruction algorithm with the power of deep learning, DeepRecon Pro can convert a collection of high-noise 2D projection images into a single 3D volume that accurately represents the object being studied. It produces this improved microscope image quality with an order of magnitude improvement in throughput. This result is achieved using deep convolutional neural networks and multiple physical noise realizations of the data. Instead of training with “one-size-fits-all” general network models, for each new sample class and acquisition geometry a separate DeepRecon Pro model can be optimally trained. This avoids the need to collect and curate a large training dataset, training times are shorter, and the trained model is a significantly better fit to the sample type and acquisition geometry. The solution was implemented as a single-click workflow so that users with no experience in deep learning can use DeepRecon Pro out of the box. Unlike other deep learning technologies that require laborious parameter tuning and manual dataset annotation, DeepRecon Pro greatly simplifies the training process and makes it virtually parameter-free, resulting in near-perfect denoising under almost any condition. It also requires nothing more than a desktop-class workstation and is able to produce images of better quality in less time than traditional iterative reconstruction algorithms.
X-ray microscopy allows non-destructive imaging of the interior of almost anything from a car battery to a meteorite. Unfortunately, X-ray microscopy typically suffers from a severe trade-off: for its high spatial resolution (several orders of magnitude better than clinical CT), the field-of-view is small and the acquisition time is long. DeepRecon Pro shifts the trade-off toward faster scans while still maintaining image quality.
With its reduced scan times and improved image quality, DeepRecon Pro can perform in situ microscopy with scan times much closer to the dynamics of various processes, for example, charge cycling of batteries. Scan time reduction may improve in-line inspection of semiconductors. Improved image quality may be used to develop critical physics-based models for geological materials and the brain tissue of vertebrates.
NL5 for Fast Live-Cell Imaging
Confocal.nl
Developers: Stefan Lampaert and Erik Manders

The NL5 is a line-scan confocal upgrade that turns virtually any research microscope into a fast confocal imaging system with high resolution and sensitivity. A slit pinhole, together with a sensitive sCMOS camera and fast scanner technology, makes the NL5 a new tool for 3D live cell imaging in four colors. Conventional pinhole confocal microscopes employing point-scanning are limited in imaging speed. However, the slit pinhole allows the NL5 to scan a full line at a time instead of just a point, increasing the acquisition speed, which in turn significantly shortens laser light exposure, which normally would produce photobleaching and phototoxicity. Combining the slit pinhole of the NL5 with a sensitive sCMOS camera operating at 25 fps increases imaging sensitivity further so the laser may be operated at lower power. The result is the capture of high signal-to-noise ratio images without signs of phototoxicity or bleaching.
The main competition to the NL5 in terms of acquisition speed is the spinning-disk system. But contrary to spinning-disk systems, the NL5 with its slit pinhole suffers from less pinhole crosstalk when imaging deep in a sample. The NL5 is capable of raw image resolution of 240 nm, or 170 nm after deconvolution. Conventional confocal microscopes are limited to imaging speeds on the order of 5 seconds per image, assuming Nyquist-criterion scanning. The NL5 produces images more than 100 times faster. This fast scanning combined with sensitive sCMOS detection permits use of low light levels for imaging live cells in long experiments.
High image quality and high acquisition speed are desired in order not to miss details of changes taking place in the sample. The NL5 technology makes it possible to image a living biological sample in 3D for a long period without damaging the sample with excitation light. This allows long-term observation of organoids and model organisms such as zebrafish. It also permits 3D visualization of dynamic processes in live cells such as mitochondrial dynamics, mitosis analysis, and cell division tracking. Besides fast-scanning capability, the NL5 provides high contrast in 3D imaging deep into the specimen.
DeepSIM Super-Resolution Module
CrestOptics S.p.A.
Developers: Raino Ceccarelli and Emanuele Pontecorvo

DeepSIM is a super-resolution module, based on a lattice multi-point structured illumination microscopy (SIM) system, compatible with any existing upright or inverted microscope with a camera port. The DeepSIM super-resolution module can be integrated either as a standalone instrument or in combination with an X-light V3 spinning-disk setup, allowing seamless changes from wide-field to confocal and confocal to super-resolution. The working principle is straightforward: laser light enters the DeepSIM via a multi-mode fiber and is focused through the pinholes of a mask of micro lenses; the resulting lattice pattern is projected onto the sample via a motorized galvo. Emission light coming from the sample goes through a dichroic mirror and emission filters, then the selected emission frequency is detected by the camera. The DeepSIM is a reliable, simple-to-use, and affordable solution for the study of cellular structures up to an XY resolution of ~100 nm without the need to apply any special sample preparation protocol. Deep data can be obtained in the following ways: super-resolved optical sectioning with Z resolution up to ~300 nm; dynamic processes in deep specimens with a temporal resolution greater than 10 fps (1024 × 1024); and deep imaging to 50 μm depths for non-clarified samples and up to 100 μm in cleared samples.
Lattice SIM using multi-point patterns rather than grid lines is shifted in a single direction, without rotations. In this manner, light and time are more efficiently used, enabling fast and gentle 3D super-resolution imaging. The multi-point pattern also offers higher contrast in deep samples making image reconstruction more robust against artifacts.
Super-resolution microscopy has become an increasingly popular and disruptive tool across the life sciences to study minute cellular structures and processes. However, due to its cost and complexity, it is not yet routine in typical laboratories. To make super-resolution accessible for all scientists, the DeepSIM has a favorable cost-efficiency ratio, universal compatibility, and modular design. DeepSIM brings out highly resolved details in conventionally prepared specimens using widely available objectives. The use of the DeepSIM with low-magnification objectives brings the benefits of an air lens: no need for immersion media or a greater working distance.
FAST-EM Multibeam Scanning Electron Microscope
Delmic
Developers: Sander den Hoedt, Andries Effting, Wilco Zuidema, Thera Pals, Sabrina Rossberger, Remco Jager, Marc van Eert, Robert Wassens, Jens Greiser, Bohuslav Seďa, Radim Šejnoha, and Pieter Kruit

FAST-EM is a multibeam scanning electron microscope with 64 parallel beams and dedicated data management that can acquire large field-of-view (FV) images at 4 nm resolution 100 times faster than standard SEM microscopes. FAST-EM is equipped with a patent-protected multibeam STEM detection system that offers superior image quality at short dwell times. The FAST-EM acquires images rapidly in transmission mode with more automation than any single-beam system (either SEM or TEM). FAST-EM can operate unattended up to 3 days and can store data locally up to 200 TB.
The 64 parallel beams impinge on a thin slice of biological material, go through it, and then excite a rigid scintillator acting as the sample substrate. Light emitted from the scintillator is inversely proportional to the density of the biological material above it. This light is then transported out of the vacuum chamber to a silicon photomultiplier array that is responsible for image formation. The system continuously monitors the quality of the 64 beamlets by observing them with a diagnostic camera and automatically adjusting focus, stigmation, and alignment as required during unattended data acquisition.
The typical settings of the acquisition system are such that images are obtained with a 4 nm pixel size, and a field of 6400 × 6400 pixels contains 41 megapixels. Fields are automatically stitched together to obtain seamless “megafields,” final images that provide both detail and context. With its rigid substrates, FAST-EM is compatible with most sample collection methods, even automated ones. Thousands of sections can be collected and imaged at high speed.
FAST-EM offers the possibility to observe the ultrastructural details of tissue and cells, as well as the context in which certain phenomena take place. Large fields-of-view can be acquired without compromising resolution, which enables better statistical descriptions of the phenomena of interest. Furthermore, biological phenomena happen in 3D. Obtaining 3D visualizations of biological material requires imaging of sequential 2D sections and stacking them together. The faster, more automated workflow offered by FAST-EM transforms electron microscopy into a powerful quantitative technique that significantly reduces the time required to obtain 2D images and 3D reconstructions of biological phenomena.
VistaScope Broadband Multiphoton Microscope
Scientifica, Ltd.
Developers: Kelly Sakaki and Roland Hadleigh

The VistaScope is a multiphoton laser-scanning microscope developed for minimally invasive sub-micron-resolution imaging of large volumes in biological tissue. The most important advance is increased field-of-view (FOV), more than doubling the specimen area in a single image when compared to the previous de facto industry standard. This is achieved with no compromise on the obtainable microscope resolution or evenness of illumination. The next advance is in the excitation bandwidth, providing full wavelength coverage (700–1700 nm) with a single lens design, enabling diffraction-limited imaging across the full two- and three-photon wavelength range on the same microscope. For example, two-, three-, and four-photon microscopy of DAPI id possible. The VistaScope provides two identical independent laser scanning paths, both of which can be used for imaging or photostimulation. The paths are combined using polarization, allowing full wavelength independence.
Imaging large volumes at scale and with sufficient time resolution requires a throughput of more than a cubic-millimeter per minute at sub-micron lateral resolution. The VistaScan large FOV achieves this throughput; for example, the specimen area is 1.8 × 1.8 mm2 when using an off-the-shelf 16× objective which increases to 2.9 × 2.9 mm2 with 10× objectives. This imaging is further enhanced by even illumination across the entire FOV (less than 10% variation), which is the result of using a fully relayed scanning system and imaging each scanning mirror onto the next. This homogeneous illumination ensures that data quality is consistent over the entire FOV, even when approaching the edges.
The versatility of the VistaScope is further enhanced since it is built on the same mechanical platform as Scientifica's HyperScope and is compatible with all extensions available for HyperScope. The shared mechanical platform also means that any existing or future HyperScope system will be field-upgradable to a VistaScope, bringing the next generation imaging capabilities to existing users at roughly a quarter of the price of a completely new microscope.
The functionality of the VistaScope is geared toward neuroscience research. The ability to combine two-photon and three-photon imaging with large FOV allows the analysis of activity in different brain regions for a range of model organisms. The ability to drive the two scan paths independently to simultaneously image distinct regions of the brain and apply temporal demultiplexing to the fluorescence signal allows studies of neuronal integration in real time. The large FOV size and wide wavelength coverage are also applicable to intravital imaging of organ systems such as lung, kidney, and gut.
ARPAS Measures Atom Acidity
TU Wien, FAU Erlangen-Nürnberg, and Charles University
Developers: Margareta Wagner, Bernd Meyer, Martin Setvín, Michael Schmid, and Ulrike Diebold

Atomically-resolved determination of proton affinity of surface atoms (ARPAS) is a method to quantitatively determine the acidity of individual surface atoms of a solid using non-contact atomic force microscopy (AFM). The acidity of solids is a key fundamental quantity that determines their behavior in many applications, from catalysis to dissolution processes. For molecules, acidity is well-defined since each acidic atom has the same environment. This is not true for solid surfaces where each atom can have a different acidity depending on its environment. ARPAS determines the acidity of individual atoms on solid surfaces using measurements of force-distance curves on individual surface hydroxyls that are first identified in atomically-resolved images. The AFM tip is tungsten-coated with indium oxide. Experimental force-distance curves are reliable and reproducible, and the measured forces can be calibrated with ab initio calculations on model molecules. Experimentally, these F(z) curves are obtained with a low-temperature nc-AFM that can provide atomic resolution and site-specific measurements with an OH-functionalized tip. The interaction strength between the tip and any atom on the surface (maximum attractive force) is related to the proton affinity of the surface atom, which is linearly related to the acidity of the respective OH groups. To calibrate the experimental forces, density function theory (DFT) calculations modeling the functionalized AFM tip are applied to test molecules with known gas-phase acidities (proton affinities) to calculate the maximum attractive forces.
The ARPAS AFM method is a radically new approach. Never before has it been possible to assess a fundamental property of a single atom on a surface quantitatively. The surfaces of solids are notoriously heterogeneous, and the properties of the same type of atom can be very different depending on its neighborhood. Acidity could be computed theoretically, but it was impossible to compare with experiment since all other methods assessing acidity of a solid surface are area-averaging, that is, they measure all the different atoms at once.
Initially, ARPAS will be used mainly to provide benchmark data on various sample surfaces and for atoms next to defects, dopants, steps, or other adsorbates. Eventually the method might assess and tune the properties of solid surfaces atom-by-atom.