Introduction
Storage entomology is important in India for managing stored grains because it prevents significant yield losses caused by pests during storage. In India, 60–65% (about 170–175 million tonnes) of agricultural products are stored by farmers themselves in gunny bags or traditional storage structures, allowing pests to easily infest the grain. Annual storage losses in India have been estimated to be around 14 000 000 tonnes, valued at 7000 crore (∼ $US 1.75 billion), with insects alone accounting for 1300 crore (∼ $US 325 million) of the loss (Indian Grain Storage Management and Research Institute 2019). Approximately 800 insect species have been linked to stored products worldwide. Around 100 insect and mite species are associated with stored grains in India. Thirty species are regarded as important pests, and they are known to cause significant quantitative and qualitative losses in retail products. Coleopterans are the most numerous, with approximately 17 regular important species known to infest stored goods (Prakash et al. Reference Prakash, Rao, Sahoo, Kishore, Singh and Jamir2016; Tyagi et al. Reference Tyagi, Guru, Nimesh, Bashir, Patgiri, Mohod and Khatkar2019). The red flour beetle, Tribolium castaneum Herbst (Coleoptera: Tenebrionidae), is the most prevalent pest of stored goods. It has the highest fecundity rate of any stored product insect and causes significant harm to stored grains. As a result, grain protection demands significant attention for higher output, and after-harvest losses are directly related to a country’s lack of economic progress.
Fumigation plays an important role in eradicating pests from stored goods. Synthetic fumigant is commonly used to protect stored grains from pests, and the continuous use of various pesticides has resulted in risks such as pesticide resistance, contamination of stored products with toxic residues, handling hazards, increased application costs, and environmental impacts (Kumar Reference Kumar2012). These concerning issues drive the need for environmentally friendly and biodegradable management options. Botanicals are one of the safest pesticides to employ because they have a broad spectrum of activity, demonstrate minimal toxicity against mammals, and are easier to prepare and use at the farm level. Aromatic herbs and their essential oils have been employed as effective bio-pesticides to control the stored-grain pest in recent years. Tea tree, Melaleuca alternifolia Cheel (Myrtaceae), has been used to treat acne, sore throats, wounds, coughs, and colds for centuries (Shemesh and Mayo Reference Shemesh and Mayo1991). In laboratory trials, M. alternifolia oil has been found to have antibacterial, antifungal, antiviral, antiprotozoal, anti-inflammatory, and insecticidal activities (Carson et al. Reference Carson, Hammer and Riley2006; Hammer et al. Reference Hammer, Carson, Riley and Nielsen2006).
Insect detoxification enzymes such as esterases, glutathione-S-transferases, and cytochrome P450s play vital roles in the resistance and metabolism of insecticides in insects. The increased activity of carboxylesterase, glutathione-S-transferase, and P450 enzymes resulting from the induction of xenobiotics would decrease insecticide toxicity because of enhanced metabolism. The insensitivity of these target sites to pesticides is usually related to the activity of acetylcholinesterase. Phosphatase enzymes are also considered as resistance-causing agents against carbamates and organophosphate insecticides (Gong et al. Reference Gong, Wang, Shi, Kang, Zhu and Jin2013; Simon Reference Simon2015). These are the major marker enzymes used to study insecticide resistance development in insects. Some chemical agents are able to create free radicals that cause DNA damage. If pests fail to repair the damaged DNA, it leads to death. A simple method to identify DNA damage in pests is the comet assay, based on the detection of broken strands of DNA and alkali label sites by measuring the DNA migration from the nuclear DNA (Qari et al. Reference Qari, Abdel-Fattah and Shehawy2017; Tice et al. Reference Tice, Agurell, Anderson, Burlinson, Hartmann and Kobayashi2000). The purpose of the present study was to determine the insecticidal activity of M. alternifolia essential oil against T. castaneum and the potential inhibitory effects on the development of insecticide resistance through enzymatic activity.
Materials and methods
Insect culture and essential oil
Tribolium castaneum culture was maintained in the Insectarium, Department of Zoology, University of Madras (Chennai, Tamil Nadu, India; 13.01140° N, 80.24006° E). The culture was kept in a plastic container (30 × 20 cm) with wheat flour at room temperature 27 ± 2 °C and a 65–75% relative humidity. Each container was maintained with 200 insects, and no cannibalism occurred.
The essential oil of M. alternifolia was purchased from the Government of Tamil Nadu’s Medicinal Plant Development Area, Doddabetta (Tamil Nadu, India).
Determination of tea tree oil toxicity (LC50)
The toxicity of tea tree oil against T. castaneum was investigated using a soaked-filter paper technique (Negahban et al. Reference Negahban, Moharramipour and Sefidkon2007). Adults (2–4 days old) and third-instar larvae were used in the experiment. Tribolium castaneum has six larval instars. The first- and second-instar larvae are tiny and fragile to handle; because of this, we used third-instar larvae for the toxicity study. Fumigation bioassay concentrations were determined from the preliminary screening. For preliminary screening, different concentrations ranging from 10 to 900 µL/L were used to identify the effective concentrations against adults and larvae.
Adults and larvae were treated with different concentrations of the essential oil, ranging from 40 to 500 µL/L. To prevent the insects from directly coming into contact with the essential oil, Whatman No.1 filter paper (2 cm diameter) was soaked with oil at the desired concentration and then was affixed under the lids of 100-mL glass containers (7.0 cm height × 3.5 cm diameter) with nylon net. Ten insects (adult and larvae) were released into each container, 2 g of feed were placed inside each container, and the lids closed tightly to prevent air flow. Each essential oil concentration was replicated five times. Adult mortality was assessed at 6, 12, and 24 hours after treatment. Larval mortality was assessed at 12, 24, and 48 hours after treatment. Controls consisted of similar set-ups without essential oil. The sublethal and lethal concentrations of essential oil were calculated using IBM SPSS software, version 25 (IBM, Armonk, New York, United States of America; https://www.ibm.com/spss?utm_content=SRCWW&p1=Search&p4=43700068092266129&p5=p&gclid=CjwKCAiAkfucBhBBEiwAFjbkr1VkZOWsG4KN1No3fi3jlFxpqhowY91mC7jZ8ltuxAw8HGPp81P0ixoCHg0QAvD_BwE&gclsrc=aw.ds).
Gas chromatography–mass spectrometry
Gas chromatography–mass spectrometry analysis was performed with an Agilent-8890 (Agilent Scientific Equipment, Santa Clara, California, United States of America), coupled with an MSD 5977 mass selective detector (Agilent Scientific Equipment) operating at 70 eV energy. The 1-µL samples were injected in a split mode at a ratio of 15:1 at 11.36 psi. The compounds were separated using an HP-5MS column (30 m × 250 µm ID × 0.25 µm; Agilent Scientific Equipment), with helium being the carrier gas at an average velocity of 40.40 cm/second at a flow rate of 1.2 mL/minute. The initial temperature was 75 °C and was ramped to 180 °C at a rate of 5 °C/minute, with a hold time of 3 minutes. The final temperature of 300 °C was achieved at a rate of 5 °C/minute with a hold time of 5 minutes. The quad temperature was 150 °C, the transfer line temperature was 280 °C, and the source temperature was 230 °C. The total running time was 53.5 minutes. The solvent delay was 6 minutes, and scan-time segments were started at a mass of 50–600 m/z, with a threshold of 150 at a scan speed of 1562 m/z using the 2.3 schema version. The identification of the essential oil constituents was performed using the National Institute of Standards and Technology (NIST) 17 mass spectral library.
Quantitative analysis of biochemical constituents
Using the 50% lethal concentration (LC50) of tea tree oil as determined in the toxicity experiment, new adults were treated as described in the toxicity experiment. Ten live treated adults and ten live control adults from different jars were randomly selected for study and were homogenised with ice-cold phosphate buffer (pH 7.0) using a Teflon glass homogeniser. They then were centrifuged for 20 minutes at 21 918 g at 4 °C. The supernatant was stored at –20 °C until required for further analysis.
Lowry et al.’s (Reference Lowry, Rosebrough, Farr and Randall1951) method was used to calculate the protein concentration. Acetylcholinesterase activity was determined using acetylthiocholine iodide as a substrate (Ellman et al. Reference Ellman, Courtney, Andres and Featherstone1961; Ikezawa and Taguchi Reference Ikezawa, Taguchi and Lowenstein1981). Carboxylesterase activity was determined using the method described by Ramachandran et al. (Reference Ramachandran, Baskar and Jayakumar2022). Levels of acid phosphatases and alkaline phosphatases were determined by using Asakura’s (Reference Asakura1978) method. Glutathione-S-transferase activity was determined using Brogdon and Barber’s (Reference Brogdon and Barber1990) method.
Qualitative analysis of biochemical constituents
Adult total protein and carboxylesterase profiles were analysed using the nondenatured discontinuous polyacrylamide gel electrophoresis. A 4% stacking gel (pH 6.8) and an 8% separating gel (pH 8.8) were used in Tris-glycine buffer (pH 8.3) for the polyacrylamide gel electrophoresis. Control and treated adult samples were electrophoresed on a slab gel at 10 °C with a constant current of 4 mA. The gel was stained after electrophoresis to enable detection of total protein and of α-carboxylesterase and β-carboxylesterase activity.
For protein visualisation, the gel was stained with Coomassie brilliant blue R-250 (SRL Chemicals, Mumbai, India). Electrophoretically separated protein bands with α-carboxylesterase and β-carboxylesterase activity were detected according to the method described by Kirkeby and Moe (Reference Kirkeby and Moe1983) and Argentine and James (Reference Argentine and James1995).
DNA fragmentation assay
Total genomic DNA was obtained using the phenol:chloroform:isoamyl alcohol technique for the DNA fragmentation assay (Sambrook et al. Reference Sambrook, Fritschi and Maniatis1989). The 50 adults that had been exposed to an LC50 concentration of essential tea tree oil and a second set of 50 control adults (i.e., that were not exposed to tea tree oil) were homogenised separately using a Teflon glass homogeniser with 500-µL digestion buffer (pH 8.0). Each of the homogenates was treated as follows: the homogenate was transferred to a sterile 2-mL Eppendorf tube with 10 µL of proteinase K (SRL Chemicals, Mumbai, India) and maintained at 55 °C for 1 hour in a thermomixer. We added 500 µL of phenol:chloroform:isoamyl alcohol (25:24:1; SRL Chemicals, Mumbai, India) and centrifuged the mixture at 8000 g for 5 minutes at 4 °C. After collecting 300 µL of supernatant, an equal volume of chloroform:isoamyl alcohol (24:1) was added and centrifuged at 8000 g for 5 minutes at 4 °C. We collected the supernatant (200 µL) in a sterile Eppendorf tube, added 100 µL of 7.5 M ammonium acetate (SRL Chemicals, Mumbai, India) and 400 µL of ice-cold ethanol, and then maintained the resulting mixture at 4 °C for 1 hour to allow the DNA to precipitate. The sample was then centrifuged for 5 minutes at 8000 g. The supernatant was removed, and the pellet was washed with ice-cold ethanol and recentrifuged for 5 minutes at 8000 g. The pellet was washed three times and set aside to dry. The pellet was then dissolved in 50 µL TE (Tris + EDTA) buffer (SRL Chemicals, Mumbai, India) and kept at 4 °C for further use. Fragmentation of DNA was determined using a 1% agarose gel electrophoresis at 50 V in 4 °C for both the control and treatment DNA samples.
Alkaline single cell gel electrophoresis: comet assay
Assessment of DNA damage in individual cells of T. castaneum was analysed using a comet assay (Singh et al. Reference Singh, McCoy, Tice and Schneider1988). The 50 treated (LC50) and 50 control live adults were held at 0 °C for 40 seconds to immobilise them before each group was homogenised separately with 1 mL of 100 mM phosphate buffer saline (pH 7.5; SRL Chemicals, Mumbai, India) using a Teflon glass homogeniser. Each homogenate was filtered through a 125-µm nylon mesh before being used for further study.
The sterile slides were first coated with 1% low melting point agarose (SRL Chemicals, Mumbai, India), then with 0.5% low melting point agarose-containing cells (1 × 104), and then with a final coating of 1% low melting point agarose. The precoated slides were kept in lysis solution (pH 10; 2.5 M NaCl, 100 mM Na2EDTA, 10 mM Tris, 10% DMSO, 1% Sarkosyl, and 1% Triton X-100) at 4 °C for 1 hour to remove lysed proteins from cells. The slides were then placed in a horizontal gel electrophoresis platform at 4 °C for 20 minutes, covered with ice-cold TAE (Tris + acetic acid + EDTA) buffer (pH 13), and electrophoresis was performed at 25 V, 300 mA. The electrophoresed slides were immersed in neutralising buffer three times for 5 minutes each (0.4 M Tris–HCl, pH 7.5). After that, the slides were dehydrated for 5 minutes with ethanol and stained with ethidium bromide. To remove excess stain, the slides were dipped in sterile double-distilled water and covered with a cover slip before being examined under a fluorescent microscope to observe DNA damage. To avoid any DNA damage that might be caused by white light, all of the preceding steps were carried out in low light.
During electrophoresis, broken DNA fragments (tail) migrate from the super-coiled DNA (head). After staining, the DNA resembles a comet with bright fluorescing heads and tails, the length and intensity of which are determined by the level of DNA-strand breakage within the cells. The tail length and movement were used as an indicator of DNA damage. Cell damage was graded according to Mitchelmore and Chipman’s (Reference Mitchelmore and Chipman1998) scale: 0 to minimal damage: 5%; low damage: 5–20%; moderate damage: 20–40%; high damage: 40–75%; and extreme damage: 75–100%.
Statistical analysis
Data from the determination of the tea tree oil LC50 experiment were subject to probit analysis with 95% confidence limits. One-way analysis of variance with a post-hoc Tukey’s test was used to examine the significant differences between treatments (P < 0.05). All above statistical analyses were performed using IBM’s SPSS software, version 25 (https://www.ibm.com/spss?utm_content=SRCWW&p1=Search&p4=43700068092266129&p5=p&gclid=CjwKCAiAkfucBhBBEiwAFjbkr1VkZOWsG4KN1No3fi3jlFxpqhowY91mC7jZ8ltuxAw8HGPp81P0ixoCHg0QAvD_BwE&gclsrc=aw.ds). For gel band quantification, Vilber’s Quantum Bio-vision programme (Vilber, Marne-la-Vallée, France) was used. CASP software (1.2.3, beta 2 version; CASP, Athens, Greece) was used to calculate the percentage of DNA damage.
Results
Determination of tea tree oil LC50
After 24 hours of exposure to M. alternifolia essential oil, the greatest mortality of adults occurred at 200 µL/L (100%; Table 1). The lethal concentration of M. alternifolia essential oil against adults was LC50 122.7 µL/L and LC90 180.1 µL/L. The fumigation toxicity of M. alternifolia essential oil on larvae showed maximum 96% mortality at 500 µL/L after 48 hours of exposure (Table 2). The lethal concentration of larvae was LC50 280.01 µL/L and LC90 541.47 µL/L (Table 3). No mortality was observed in the controls.
Table 1. Mean (+/– standard error) percent mortality of T. castaneum adults when exposed to various concentrations of M. alternifolia essential oil.

Mean of five replication ± standard error. The letters within column denote significant differences by Tukey’s test (P ≤ 0.05).
Table 2. Mean (+/– standard error) percent mortality of T. castaneum larvae when exposed to various concentrations of M. alternifolia essential oil.

Mean of five replication ± standard error. The letters within column denote significant differences by Tukey’s test (P ≤ 0.05).
Table 3. LC50 of M. alternifolia against T. castaneum adults and larvae.

* LC50 calculated from raw mortality data using SPSS software, version 25.
The gas chromatography–mass spectrometry analysis of M. alternifolia essential oil showed a total of 20 peaks (Fig. 1). The primary compound was terpinen-4-ol (31.78%), followed by α-terpineol (20.24%) and terpinolene (17.94%; Table 4).
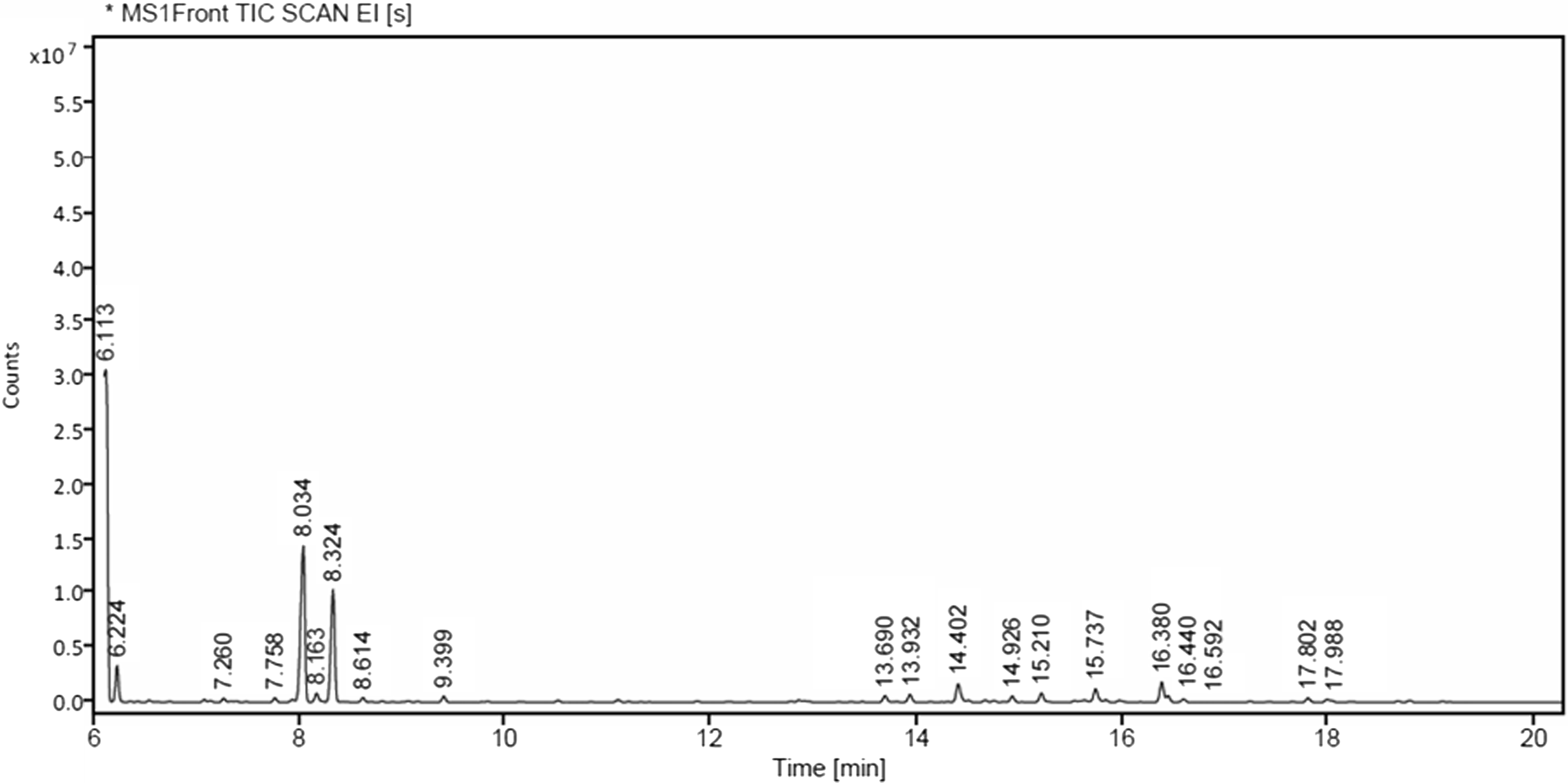
Fig. 1. Gas chromatography–mass spectrometry chromatogram of tea tree oil.
Table 4. Volatile compositions of M. alternifolia essential oil.

Quantitative analysis of biochemical constituents
The lethal concentration of M. alternifolia essential oil significantly reduced the total protein content of the treated T. castaneum adults compared to that of the control adults (Table 5). The total protein content of control and treated adults was 7.41 and 6.22 µg/µL, respectively. Acetylcholinesterase activity was also reduced in treated adults compared to that in the control adults (3.2 and 3.07 µM AcT hydrolysed/minute/mg of protein). Both α-carboxylesterase activity and β-carboxylesterase activity were significantly decreased in treated adults compared to that activity in the control adults. Activity of α-carboxylesterase was 88.9 µM (control) and 67.24 µM (treated) of α-naphthol released/minute/mg of protein; activity of β-carboxylesterase was 0.164 µM (control) and 0.06 µM (treated) of β-naphthol released/minute/mg of protein. No significant differences in acid and alkaline phosphate activity were detected between the treated and control groups. Glutathione-S-transferase activity was significantly increased in the treated adults compared to in the controls: 0.36 and 0.21 1-chloro-2,4-dinitrobenzene product/minute/mg of protein (Table 5).
Table 5. Percent (+/– standard error) mortality of sublethal concentrations of M. alternifolia oil on enzyme activity of T. castaneum adults. N = 3.

* Significant difference when compared against the control.
Qualitative analysis of biochemical constituents
The protein bands were quantitatively analysed by native polyacrylamide gel electrophoresis, and the intensity of bands was measured by densitometer using Bio-vision software (Vilber). For total protein, two bands appeared in the gels for each of the treated and control groups. The intensity of the first band was decreased by 11.3% in the treated group. The second band showed increased intensity by about 2.9% in the treatment group compared to that of the control group (Fig. 2A).

Fig. 2. Qualitative analysis of biochemical components of whole body homogenate of Tribolium castaneum adult by native polyacrylamide gel electrophoresis: A, total protein; B, α-carboxylesterase; and C, β-carboxylesterase.
The α-carboxylesterase-stained gel revealed the presence of five bands in the control group. In the treated group, bands 3 and 4 had disappeared completely (indicated by arrows in Fig. 2B). The intensity of bands 1 and 5 showed a decrease of 70.7% and 25.7%, respectively. The intensity of band 2 showed an increase of 71.2% in the treatment group compared to that in the control group. Six isoenzyme bands were detected in the control group for β-carboxylesterase. In the treatment group, bands 4 and 5 completely disappeared. The intensity of bands 1 and 3 showed an increase of about 8.6% and 84.42% in the treatment group and control group, respectively (Fig. 2C). When compared with bands 2 and 6 in the control group, the intensity of bands 2 and 6 in the treatment group was reduced by about 78.6% and 37.9%, respectively.
DNA fragmentation and comet assay
Some DNA fragmentation was found to occur in adult beetles treated with the LC50 dose of tea tree oil. Agarose gel electrophoresis showed smeared DNA fragments between 600 and 350 bp (a 100-bp DNA ladder was used; Fig. 3).

Fig. 3. Detection of DNA fragmentation of Tribolium castaneum adult by agarose gel electrophoresis.
The comet assay revealed DNA damage in LC50-treated adults. The mean tail area (285.19%), tail DNA length (13.25 µM), tail DNA percentage (8.45%), and olive tail moment (1.15 µM) were all increased in treated adults compared to in the control group, which showed the following measures: mean tail area (7.32%), tail DNA length (0.26 µM), tail DNA percentage (0.12%), and olive tail moment (0.004 µM; Table 6).
Table 6. Mean (+/– standard error) percent cell damage of T. castaneum adults exposed to M. alternifolia at a sublethal concentration.

Discussion
Toxic fumigants can be an effective treatment to control stored-grain pests. In the present study, M. alternifolia essential oil showed lethal toxicity when used as a fumigant against both adults and larvae of T. castaneum. Our results show that the insect’s adult life stage is more susceptible than the larval stage. Similarly, when Wang et al. (Reference Wang, Zhu, Zhou, Niu and Lei2006) evaluated the effectiveness of fumigant Artemisia vulgaris Linnaeus (Asteraceae) essential oil against T. castaneum, 100% mortality of adults was observed at 8 µL/mL but only 49–52% mortality of larvae occurred at same dose. The volatile compounds present in essential oil likely enter into the insect through its respiratory system: an insects’ spiracles, located on the lateral surface of their body, are a likely entry point for fumigants. It has been shown that some gases may diffuse through the integument of insects, but the entry route of fumigants remains unknown at this time (Bond Reference Bond1984).
The chemical composition of an essential oil may vary according to where and when the plant is grown. Noumi et al. (Reference Noumi, Snoussi, Hajlaoui, Trabelsi, Ksouri, Valentin and Bakhrouf2011) showed that M. alternifolia essential oil from France contained 40.44% terpinen-4-ol, 3.31% α-terpineol, and 3.09% α-terpinolene. In the present study, the composition percentage of compounds in M. alternifolia from India contained similar levels of terpinen-4-ol (31.78%) but higher levels of α-terpineol (20.24%) and terpinolene (17.94%).
When an insect is treated with essential oils, protein synthesis may be blocked at the cellular level and catabolism processes may increase, resulting in low availability of total protein content. Similar protein reductions in treated insects were found by Upadhyay et al. (Reference Upadhyay, Yadav and Ahmad2011), who evaluated the biochemical parameters of T. castaneum against Capparis decidua Linnaeus (Capparaceae) and found a reduction of protein content of up to 54.85% compared to in controls.
Acetylcholinesterase is associated with cholinergic synapses, where it rapidly terminates the influx transmission by hydrolysing acetylcholine. The inhibition of acetylcholinesterase causes a desensitisation of the acetylcholine receptor and leads to obstruction of the influx transmission (Fournier et al. Reference Fournier, Bride, Hoffmann and Karch1992). Mishra et al. (Reference Mishra, Tripathi and Tripathi2014) studied the acetylcholinesterase activity of T. castaneum against Citrus reticulata Linnaeus (Rutaceae) and Mentha arvensis Linnaeus (Lamiaceae) essential oils at LC50 doses. Acetylcholinesterase activity was reduced as a result of both essential oil treatments: 0.049 µM of substrate hydrolysed min−1 mg−1 of protein with C. reticulata and 0.054 µM of substrate hydrolysed min−1 mg−1 of protein with M. arvensis, compared to 0.080 µM of substrate hydrolysed min−1 mg−1 of protein in the control. Melaleuca alternifolia essential oil treatment against T. castaneum significantly inhibited the activity of α-carboxylesterase, β-carboxylesterase, and glutathione-S-transferase enzymes. In parallel, an LC50 dose of Artemisia dracunculus against T. castaneum reduced the levels of carboxylesterase and glutathione-S-transferase enzymes in the insect (Shojaei et al. Reference Shojaei, Talebi, Sharifian and Ahsaei2017). Resistance in insects is a complex phenomenon, often involving more than one mechanism, including, enhanced enzymatic metabolism, altered target site sensitivity, penetration resistance, and altered behavioural patterns. Carboxylesterase and glutathione-S-transferase are major enzymes that can play vital roles in resistance development through the degradation of the insecticide molecule. In the present study, carboxylesterase activity was reduced by treatment with M. alternifolia compared to what was observed in the control, revealing that the essential oil could interrupt normal production enzymes and may not be degraded.
Adults of T. castaneum treated with the LC50 dose of M. alternifolia essential oil showed a wide variation in protein, α- carboxylesterase, and β-carboxylesterase profiles, as revealed via native polyacrylamide gel electrophoresis. In a similar study, Al Qahtani et al. (Reference Al Qahtani, Al-Dhafar and Rady2012) evaluated the toxicity of Zingiber officinale Roscoe (Zingiberaceae), Elettaria cardamomum Linnaeus (Zingiberaceae), and Foeniculum vulgare Miller (Apiaceae) against Oryzaephilus surinamensis (Coleoptera: Silvanidae). The protein profiles of the treated insects were altered when compared to those in the control. A treatment of Annona muricata Linnaeus (Annonaceae) saline extract on Aedes aegypti (Diptera: Culicidae) larvae altered the intensity of carboxylesterase bands (Parthiban et al. Reference Parthiban, Arokiyaraj and Ramanibai2020). The DNA fragmentation and comet assay results in that study revealed the presence of DNA damage in treated adults. These results are supported by Qari et al. (Reference Qari, Abdel-Fattah and Shehawy2017), who evaluated the DNA damage of Rhyzopertha dominica (Coleoptera: Bostrichidae) adults against various essential oils. Maximum DNA damage occurred in an Origanum majorana Linnaeus (Lamiaceae) essential oil treatment, at 42.74% compared to damage observed in the control (8.3%).
Conclusion
The present study provides promising results for the control of T. castaneum using M. alternifolia essential oil. The oil was shown to affect the biochemical and physiological systems in the adult beetles. This work was conducted in the laboratory; field trials would be necessary to demonstrate efficacy before industry would consider adopting this treatment.
Acknowledgements
The authors are deeply grateful to the Department of Science and Technology, New Delhi, India, and the Sophisticated Analytical Instrumentation Centre, Indian Institute of Technology, Madras, Tamil Nadu, India, for providing the service of the gas chromatography–mass spectrometry instrument. The authors also thank the Department of Zoology, University of Madras, Chennai, India, for providing necessary lab facilities.
Funding
This research did not receive any specific grant from funding agencies in the public, commercial, or not-for-profit sectors.
Conflict of interest disclosure
The authors declare they have no conflicts of interest.