1. Introduction
The Upper Triassic–lower Middle Jurassic Shemshak Group represents an important regional tectono-stratigraphic megacycle and extends across large parts of Iran (Fürsich et al. Reference Fürsich, Wilmsen, Seyed-Emami, Majidifard, Brunet, Wilmsen and Granath2009 a, b; Wilmsen et al. Reference Wilmsen, Fürsich, Seyed-Emami, Majidifard and Taheri2009 a). The Shemshak Group has been studied in different parts of Iran such as on the structurally separated, fault-bounded blocks of east-central Iran (Fürsich et al. Reference Fürsich, Hautmann, Senowbari-Daryan and Seyed-Emami2005 a; Wilmsen et al. Reference Wilmsen, Fürsich, Seyed-Emami, Majidifard, Brunet, Wilmsen and Granath2009 b; Salehi et al. Reference Salehi, Moussavi-Harami, Mahboubi, Wilmsen and Heubeck2014, Reference Salehi, Moussavi-Harami, Mahboubi, Fürsich, Wilmsen and Heubeck2018) as well as in the Alborz (Fürsich et al. Reference Fürsich, Wilmsen, Seyed-Emami, Cecca and Majidifard2005 b, Reference Fürsich, Wilmsen, Seyed-Emami, Majidifard, Brunet, Wilmsen and Granath2009 b) and Binalud Mountains (Wilmsen et al. Reference Wilmsen, Fürsich, Taheri, Brunet, Wilmsen and Granath2009 c) of north-northeast Iran. The previous studies improved our understanding of the major Cimmerian tectonic events and their regional significance (e.g. Fürsich et al. Reference Fürsich, Wilmsen, Seyed-Emami, Majidifard, Brunet, Wilmsen and Granath2009 a; Wilmsen et al. Reference Wilmsen, Fürsich, Seyed-Emami, Majidifard and Taheri2009 a), and also elucidated the shared Mesozoic geodynamic history of the larger area including the Afghan–Tajik basins (Fürsich et al. Reference Fürsich, Brunet, Auxiètre, Munsch, Brunet, McCann and Sobel2017). Within this context, the lower Middle Jurassic Hojedk Formation of the uppermost Shemshak Group of east-central Iran has been postulated to indicate inter-regional tectonic instability, uplift and erosion due to syn-depositional block-faulting (Fürsich et al. Reference Fürsich, Wilmsen, Seyed-Emami, Majidifard, Brunet, Wilmsen and Granath2009 a), shown by the vast thicknesses as well as rapid lateral facies and thickness variations in different parts of east-central Iran, including the northern Tabas Block (Wilmsen et al. Reference Wilmsen, Fürsich, Seyed-Emami, Majidifard, Brunet, Wilmsen and Granath2009 b). This essentially early Bajocian unit also reflects a short-term subsidence pulse and was deposited in c. 1.5 Ma on the way to the Mid-Cimmerian tectonic event (Salehi et al. Reference Salehi, Moussavi-Harami, Mahboubi, Fürsich, Wilmsen and Heubeck2018), which is reflected by a conspicuous unconformity surface associated with regional block-faulting and folding as well as widespread erosion in different parts of Iran (Wilmsen et al. Reference Wilmsen, Fürsich and Seyed-Emami2003, Reference Wilmsen, Fürsich, Seyed-Emami, Majidifard, Brunet, Wilmsen and Granath2009 b; Fürsich et al. Reference Fürsich, Wilmsen, Seyed-Emami, Majidifard, Brunet, Wilmsen and Granath2009 a), followed by widespread post-Mid-Cimmerian exhumation in Central Iran (Gyomlai et al. Reference Gyomlai, Agard, Jolivet, Larvet, Bonnet, Omrani, Larson, Caron and Noël2022). However, despite its obvious tectono-stratigraphic significance, the Hojedk Formation has received little attention with respect to detailed facies analyses and palaeogeographic reconstructions, in marked contrast to the underlying siliciclastic successions of the Upper Triassic and Lower Jurassic part of the Shemshak Group of east-central Iran (Nayband and Ab-e-Haji formations) that have been studied in considerable detail (Fürsich et al. Reference Fürsich, Hautmann, Senowbari-Daryan and Seyed-Emami2005 a; Salehi et al. Reference Salehi, Moussavi-Harami, Mahboubi, Wilmsen and Heubeck2014, Reference Salehi, Moussavi-Harami, Mahboubi, Fürsich, Wilmsen and Heubeck2018; Zamanian et al. Reference Zamanian, Khanehbad, Moussavi-Harami and Mahboubi2021). On the other hand, there are numerous studies on the macrofloras and palynology of the Hojedk Formation from several locations on the Tabas Block (e.g. Hashemi-Yazdi et al. Reference Hashemi-Yazdi, Sajjadi and Hashemi2014; Vaez-Javadi, Reference Vaez-Javadi2016; Badihagh & Uhl, Reference Badihagh and Uhl2019; Badihagh et al. Reference Badihagh, Sajjadi, Farmani and Uhl2019; Khalilizadeh et al. Reference Khalilizadeh, Ghaderi, Ashouri and Zand-Moghadam2019, Reference Khalilizadeh, Ashouri, Ghaderi and Barbacka2022), providing important climatic and palaeoenvironmental information. The Middle Jurassic Hojedk Formation is of major importance for understanding the regional geodynamic and palaeoclimatic evolution of the Cimmerian terranes in east-central Iran that represent a crucial part of a larger superimposed orogenic belt that extended from the Iran Plate to southeast Asia (e.g. Stampfli & Borel, Reference Stampfli and Borel2002; Golonka et al. Reference Golonka, Krobicki, Pajak, Van Giang and Zuchiewicz2006). Thus, a detailed sedimentary facies architecture and provenance study of the Hojedk Formation will greatly enhance our understanding of its stratigraphy, palaeoenvironmental and palaeogeographical setting.
Moreover, the Hojedk Formation contains extensive coal deposits, specifically in both the southern Tabas (Ravar–Kerman area; Huber & Stöcklin Reference Huber and Stöcklin1954; Huckriede et al. Reference Huckriede, Ku Rsten and Venzlaff1962) and northern Tabas Blocks (Kalshaneh area, the focus of this study), where the succession contains workable coal seams with estimated resources of almost 2.5 million tons (A Mohammadi & H Hosseinpour, unpub. report, 2018). Thus, the petrographic study of three coal samples from the lowermost part of the Hojedk Formation is key for the depositional and post-depositional history of the succession in the northern Tabas Block that can be compared to coal-geological data from the Mazinu and Parvadeh areas (central Tabas Block; Zamansani et al. Reference Zamansani, Rajabzadeh, Littke, Zieger and Baniasad2019; Esmailpour et al. Reference Esmailpour, Alipour, Doulati Ardejani, Rodrigues and Esterle2022). The main purpose of the present contribution is (i) to document a detailed reference section of the lower Middle Jurassic Hojedk Formation by means of careful bed-by-bed logging; (ii) to define its sedimentary facies based on lithology, texture and sedimentary structures as well as on stratal geometries and surface bedding type; (iii) to group the identified facies into facies associations; (iv) to characterize the depositional environment of the Hojedk Formation; (v) to identify the source area and the source rocks of the Hojedk Formation by means of provenance analyses; and (vi) to perform coal petrography on selected samples in order to identify major maceral constituents and to obtain maturity data.
The Cimmerian terranes have been accreted to Eurasia during the Eo- and Main Cimmerian phases in the Late Triassic and across the Triassic–Jurassic boundary interval (e.g. Wilmsen et al. Reference Wilmsen, Fürsich, Seyed-Emami, Majidifard and Taheri2009 a). Nevertheless, the Jurassic sedimentary record in northern (Alborz Mountains), northeastern (Binalud and Kopet-Dagh mountains) and Central Iran indicates the lack of true consolidation of the area and the persistence of considerable tectonic instability that culminated during specific intervals, i.e. the Mid- and Late Cimmerian phases (e.g. Fürsich et al. Reference Fürsich, Wilmsen, Seyed-Emami, Majidifard, Brunet, Wilmsen and Granath2009 a; Taheri et al. Reference Taheri, Fürsich, Wilmsen, Brunet, Wilmsen and Granath2009; Wilmsen et al. Reference Wilmsen, Fürsich, Seyed-Emami and Majidifard2021). Thus, we intend to elucidate the significance of the Hojedk Formation for the geodynamic evolution of the Central-East Iranian Microcontinent (CEIM) during the early Middle Jurassic in the run-up to the Mid-Cimmerian tectonic event.
2. Geological setting and stratigraphy
The study area belongs to the NW margin of the northern Tabas Block of the CEIM (Takin, Reference Takin1972; Fig. 1a). The Tabas Block forms the central structural element of the CEIM and was, along with the other adjacent, basement fault-bounded Lut and Yazd blocks (in the east and the west), part of the Cimmerian microplate assemblage (e.g. Stampfli & Borel, Reference Stampfli and Borel2002). During the Permian, this microplate detached from Gondwana, drifted northwards while the oceanic crust of the Palaeotethys was subducted northwards and finally collided with the southern margin of Eurasia (Turan Plate) during the early Late Triassic (Stöcklin, Reference Stocklin1974; Saidi et al. Reference Saidi, Brunet and Ricou1997; Stampfli & Borel, Reference Stampfli and Borel2002; Wilmsen et al. Reference Wilmsen, Fürsich, Seyed-Emami, Majidifard and Taheri2009 a; Chu et al. Reference Chu, Wan, Allen, Chen, Lin, Talebian and Xin2021; Mazaheri-Johari et al. Reference Mazaheri-Johari, Roghi, Caggiati, Kustatscher, Ghasemi-Nejad, Zanchi and Gianolla2022). This collision resulted in the closing of the Palaeotethys and the Eo- and Main-Cimmerian orogenic events in northern and central Iran (Wilmsen et al. Reference Wilmsen, Fürsich, Seyed-Emami, Majidifard and Taheri2009 a). The history of the Upper Triassic and lowermost Jurassic of the Shemshak Group, a thick marine to continental siliciclastic succession, was influenced by these tectonic events, which included regional uplift and deformation within the foreland system across northern and central Iran (Fürsich et al. Reference Fürsich, Wilmsen, Seyed-Emami, Majidifard, Brunet, Wilmsen and Granath2009 b; Wilmsen et al. Reference Wilmsen, Fürsich, Seyed-Emami, Majidifard and Taheri2009 a; Seyed-Emami et al. Reference Seyed-Emami, Wilmsen and Fürsich2020). The Lower to lower Middle Jurassic strata of the Shemshak Group (newly proposed Ab-e-Haji Subgroup of Seyed-Emami et al. Reference Seyed-Emami, Wilmsen and Fürsich2020) developed in fast-subsiding sedimentary basins due to both the late phase of the Cimmerian Orogeny (Main Cimmerian Event of Wilmsen et al. Reference Wilmsen, Fürsich, Seyed-Emami, Majidifard and Taheri2009 a) and the subsequent zip-like opening of an extensional back-arc basin of the Neotethys in the southern Caspian area and in northeast Iran (Brunet et al. Reference Brunet, Korotaev, Ershov and Nikishin2003; Fürsich et al. Reference Fürsich, Wilmsen, Seyed-Emami, Majidifard, Brunet, Wilmsen and Granath2009 a; Taheri et al. Reference Taheri, Fürsich, Wilmsen, Brunet, Wilmsen and Granath2009; Wilmsen et al. Reference Wilmsen, Fürsich, Seyed-Emami, Majidifard and Taheri2009 a; Seyed-Emami et al. Reference Seyed-Emami, Wilmsen and Fürsich2020). The Shemshak sedimentary megacycle was terminated by the mid-Bajocian Mid-Cimmerian tectonic event that was related to the break-up unconformity of the South Caspian Basin (Brunet et al. Reference Brunet, Korotaev, Ershov and Nikishin2003; Fürsich et al. Reference Fürsich, Wilmsen, Seyed-Emami, Majidifard, Brunet, Wilmsen and Granath2009 a).

Fig. 1. Geographical and structural framework. (a) Geographic map of Iran with its major fault systems and geological subdivision based on Shuttle Radar Topography Mission (SRTM) images. The northern Tabas Block area is indicated by a rectangle (faults modified from Konon et al. Reference Konon, Nadimi, Koprianiuk, Wysocka, Szaniawski, Wyglądała, Słaby, Beygi and Barski2016). (b) Simplified location map of the northern Tabas Block and adjacent units indicating major basement faults, sub-blocks and access roads. The location of the Kalshaneh section is shown by an asterisk.
Based on global palaeogeographic reconstructions of the Middle Jurassic time interval (Scotese, Reference Scotese2014), the CEIM was located near the southern edge of Eurasia and at the northern margin of the Neotethyan Ocean at subtropical palaeo-latitudes of around 30° N (Barrier & Vrielynck, Reference Barrier and Vrielynck2008) (Fig. 2). Except for some uplifted areas of the CEIM, most parts of the Iran Plate were covered by a shallow-marine sea during the Bajocian.

Fig. 2. Global Middle Jurassic (Bajocian–Bathonian) palaeogeography (after Scotese, Reference Scotese2014). The location of the CEIM as part of the former Cimmerian continent collage, now docked to southern Eurasia, is shown.
The onset of the anticlockwise vertical axis rotation of the CEIM along block-bounding faults (Davoudzadeh et al. Reference Davoudzadeh, Soffel and Schmidt1981; Alavi et al. Reference Alavi, Vaziri, Seyed-Emami and Lasemi1997; Mattei et al. Reference Mattei, Cifelli, Muttoni and Rashid2015) occurred most probably in the Late Jurassic (Kimmeridgian). This explains the complex facies distribution and the spatio-temporal changes in the tectonic regime of the faults, and is seen as the starting signal for the Late Cimmerian tectonic event (Wilmsen et al. Reference Wilmsen, Fürsich, Seyed-Emami and Majidifard2021). This anticlockwise rotation of the CEIM comprises in total a 135° movement in two phases (latest Jurassic to Early Cretaceous and Middle–Late Miocene) in order to transfer the Yazd, Tabas and Lut blocks into their present north–south-oriented positions (Mattei et al. Reference Mattei, Cifelli, Muttoni and Rashid2015). The block rotations probably played a significant role in accommodating the Arabia–Eurasia convergence and shortening in central Iran (Mattei et al. Reference Mattei, Cifelli, Alimohammadian and Rashid2020).
This study focuses on the lower Bajocian Hojedk Formation, the uppermost unit of the newly proposed Ab-e-Haji Subgroup (Seyed-Emami et al. Reference Seyed-Emami, Wilmsen and Fürsich2020; Fig. 3). The type section of the Hojedk Formation is located near Hojedk Village, 35 km northeast of Zarand, on the southern Tabas Block, where this formation is a thick siliciclastic, mostly continental and coal-bearing succession (Huckriede et al. Reference Huckriede, Ku Rsten and Venzlaff1962). This formation is also well-developed on the northern Tabas Block and conformably overlies ammonite-bearing limestones of the Toarcian–Aalenian Badamu Formation (Seyed-Emami et al. Reference Seyed-Emami, Schairer, Fürsich, Wilmsen and Majidifard2000). Across the northern Tabas Block and beyond, an unconformity separates the Hojedk Formation of the Ab-e-Haji Subgroup, and at a larger lithostratigraphic scale the uppermost Shemshak Group, from the marine fossiliferous limestones of the upper Bajocian Parvadeh Formation (Baghamshah Subgroup of the Magu Group) (Wilmsen et al. Reference Wilmsen, Fürsich and Seyed-Emami2003, Reference Wilmsen, Fürsich, Seyed-Emami, Majidifard, Brunet, Wilmsen and Granath2009 b; Seyed-Emami et al. Reference Seyed-Emami, Wilmsen and Fürsich2020; Fig. 3). This interregional unconformity (i.e. the Mid-Cimmerian Unconformity of Seyed-Emami and Alavi-Naini, Reference Seyed-Emami and Alavi-Naini1990) is also documented in the Alborz, Binalud and Kopet-Dagh of N-NE Iran (Fürsich et al. Reference Fürsich, Wilmsen, Seyed-Emami, Majidifard, Brunet, Wilmsen and Granath2009 a; Wilmsen et al. Reference Wilmsen, Fürsich, Taheri, Brunet, Wilmsen and Granath2009 c; Seyed-Emami et al. Reference Seyed-Emami, Wilmsen and Fürsich2020). The age of the Hojedk Formation is, based on the ammonite record of the under- and overlying formations, late Early Bajocian to earliest Late Bajocian (Seyed-Emami et al. Reference Seyed-Emami, Wilmsen and Fürsich2020), indicating that this formation was deposited in a very short interval of geological time (c. 1.5 Ma according to the Geological Time Scale 2020; Hesselbo et al. Reference Hesselbo, Ogg, Ruhl, Gradstein, Ogg, Schmitz and Ogg2020). This formation is thus time-equivalent to the marginal marine and deltaic, likewise coal-bearing Dansirit Formation in the southern Alborz (Fürsich et al. Reference Fürsich, Wilmsen, Seyed-Emami, Majidifard, Brunet, Wilmsen and Granath2009 b). Both formations reflect a distinct shallowing of the depositional environments before the Mid-Cimmerian tectonic event in northern and east-central Iran (Fürsich et al. Reference Fürsich, Wilmsen, Seyed-Emami, Majidifard, Brunet, Wilmsen and Granath2009 a, b).

Fig. 3. Lithostratigraphic framework of the Middle Jurassic (Aalenian–Bathonian) succession of the northern Tabas Block, east-central Iran (modified after Wilmsen et al. Reference Wilmsen, Fürsich, Seyed-Emami, Majidifard, Brunet, Wilmsen and Granath2009 b; Seyed-Emami et al. Reference Seyed-Emami, Wilmsen and Fürsich2020).
3. Material and methods
3.a. Field work
An expanded stratigraphic section of the Middle Jurassic Hojedk Formation in the Kalshaneh area (34° 11′ 43.96″ N, 56° 39′ 56.83″ E) was measured in detail, described, sampled and logged bed-by-bed using a modified Jacob staff (Sdzuy & Monninger, Reference Sdzuy and Monninger1985). In total, 87 hand specimens including 74 sandstone, 10 shale and 3 coal samples were collected from carefully selected representative levels in the outcrop section.
Sedimentary facies were defined based on lithology, texture, sedimentary structure, geometry and surface bedding type of strata in the field. The facies analyses were done by using the modified sedimentary facies classification of Miall (Reference Miall1985, Reference Miall2014). We emphasize that this study is focused on the continental reference section of the Hojedk Formation, which constitutes the vast majority of the succession at Kalshaneh; therefore, the thin interval of marine strata at the transition to the underlying Badamu Formation is not detailed herein. Thirty-two palaeocurrent measurements were obtained at 26 levels to constrain sedimentary dispersal patterns. To remove the influence of tectonic tilting of the strata on the palaeocurrent data, the measurements were re-rotated into the former horizontal position using rose diagrams created using Stereonet 11 software (Allmendinger, Reference Allmendinger2020).
3.b. Subsurface data
Although the Kalshaneh outcrop provides an exceptional two-dimensional exposure of the sedimentary facies relationships, a core section provides fresh and continuously drilled rocks, and thus integrated surface and subsurface data complement palaeoenvironmental reconstructions (e.g. Salehi et al. Reference Salehi, Moussavi-Harami, Mahboubi and Rahimi2015; Glad et al. Reference Glad, Willumsen, Boldreel and Clemmensen2018; Wilmsen & Bansal, Reference Wilmsen and Bansal2021). To supplement the facies analysis in the Kalshaneh outcrop, a core of an exploration borehole, focused on coal seams in the lowermost part of the Hojedk Formation, near to the measured outcrop section, was incorporated into this study to better characterize the facies and their vertical relationship and lateral variations. Thus, 187.2 m of core from borehole No. 8 (BH#8) were logged and described at the core storage facility of the Kalshaneh Coal Mine in order to evaluate subsurface aspects of facies (including lithology, textures, sedimentary structures and bed contacts). The bedding dip at the drill site is c. 55° and the drill path was vertical, so the cored thickness represents apparent stratigraphic thickness. In some cases, representative core samples were slabbed to examine internal sedimentary structures.
3.c. Analytical work
The texture, fabric and framework composition were examined in 25 standard petrographic thin-sections that were prepared from selected sandstone samples. Using a Pelcon semi-automated point counter and following the Gazzi–Dickinson method (Ingersoll et al. Reference Ingersoll, Fullard, Ford, Grimm, Pickle and Sares1984), 350 grains were counted in the petrographic thin-sections of 16 medium-grained sandstones. The modal analysis was used to classify the sandstones according to a scheme proposed by Garzanti (Reference Garzanti2019). Moreover, using the measurement tool of a Keyence digital microscope, the diameters of 500 grains in five thin-section samples were also measured. Using a micro X-ray fluorescence (μ-XRF) spectrometer (Bruker M4 Tornado), five sandstone samples were also analysed for major and minor element distribution on polished rock slabs.
Small pieces of three coal samples, collected along the underground tunnel of the main workable coal seam at Kalshaneh Coal Mine, were embedded in epoxy resin, ground and polished for coal petrography and vitrinite reflectance measurements (for the sample preparation procedure see Taylor et al. Reference Taylor, Teichmüller, Davis, Diessel, Littke and Robert1998; ISO-7404-2, 2009; and for nomenclature of the organic entities see ICCP, 1998, 2001). Vitrinite reflectance (VRr) was measured in incident light using an AxioScope.A1 microscope (Zeiss) equipped with an Epiplan-NEOFLUAR 50×/0.85 oil objective. Calibration was performed using a gadolinium–gallium–garnet standard (1.714 %Ro). The results are derived from nearly 100 measurements on each sample with a standard deviation of 0.04 to 0.07 (for details of the method see ISO-7404-5, 2009; Zieger & Littke, Reference Zieger and Littke2019).
3.d. Conceptual model and modelling workflow
One-dimensional basin modelling was conducted in order to reconstruct the burial and thermal history of the Kalshaneh area in the northern Tabas Block. The basin-modelling software PetroMod (Schlumberger; Version 2019) was used to construct the conceptual model. Input data include age, thickness and lithology of all layers (present and eroded) as well as the geological events, i.e. deposition, magmatic emplacement, erosion and non-deposition were implemented based on the local geological information obtained from published literature and maps as well as from our personal observations on the stratigraphic succession in the study area. Boundary conditions, i.e. palaeo-water depth (PWD), sediment–water interface temperature (SWIT) and basal heat flow (HF), were implemented to calculate the thermal state of the region through time. PWD was inferred from general information on the depositional environment of the formations (e.g. Wilmsen et al. Reference Wilmsen, Fürsich, Seyed-Emami, Majidifard, Brunet, Wilmsen and Granath2009 b, Reference Wilmsen, Fürsich, Seyed-Emami, Majidifard and Zamani-Pedram2010). SWIT was calculated from global maps for mean temperature at sea level based on palaeolatitude and palaeo-water depth for 33° N Central Asia (Wygrala, Reference Wygrala, Mattavelli and Novelli1988). The palaeo-basal heat flow was estimated using the Mackenzie crustal model (Jarvis & Mackenzie, Reference Jarvis and McKenzie1980) and intrusion tools for magmatic activity modelling (see below) in the software. Calibration of the model (Hartkopf-Fröder et al. Reference Hartkopf-Fröder, Königshof, Littke and Schwarzbauer2015) was controlled by applying the Easy %RoDL kinetic model (Burnham, Reference Burnham2016; Schenk et al. Reference Schenk, Peters and Burnham2017) to the vitrinite reflectance data.
4. Results
4.a. Stratigraphic section
A section (800 m thick) of the Hojedk Formation has been logged in the Kalshaneh area (Kalshaneh Coal Mine), c. 80 km NW of Tabas (Fig. 1b). Structurally, this section is located on the western limb of the Kalshaneh syncline, adjacent to the Kalmard Fault, the boundary area between the Kalmard sub-block and the northern Tabas Block (Fig. 4). The grey to brownish-grey siliciclastics of the Hojedk Formation are well-exposed in this section with a N–S trend and sub-vertical bedding dipping towards east with 45–75° (Figs 4, 5a, e). The boundary of the Hojedk Formation with the underlying Badamu Formation is conformable but abrupt. The Badamu Formation consists of dark, sandy oolithic limestones, thin-bedded calcareous sandstones and light green shales with a thickness of 175–275 m. At the top, the boundary to the overlying Parvadeh Formation is developed as a subaerial unconformity, indicated by a reddish, Fe-oxide-rich sandstone bed (Fig. 5b–d).
The basal 20 m of the Hojedk Formation in the Kalshaneh section consist of brown to light grey, fine-grained sandstone and light green to yellow shales (Fig. 6). Mud clasts are present at the base of the sandstones, and hummocky cross-stratification (HCS) was observed in some beds. Marine fossils (bivalves and oyster debris) and trace fossils (e.g. Thalassinoides) are also present. The basal unit is overlain by 65 m of alternating thick-bedded, trough cross-bedded sandstones, siltstones, dark grey to blackish coaly shales and workable coal seams with a thickness of 1–2 m, appearing c. 30 m above the base of the formation (Fig. 6). This alternation is followed by a 180 m thick succession of fining-upward cycles consisting of light grey, fine-grained sandstones, siltstones, silty shales and occasional well-developed light grey to coaly shales. The sandstones show sharp bases and are lenticular in shape with a mean width of 8–10 m and an average thickness of 1 m. When interbedded with fine-grained clastic rocks, the sandstone layers contain ball-and-pillow structures at the base. Fine-grained sandstone with sigmoidal shape and lateral accretion surfaces are common in this unit. Mud chips, plant debris and tree trunks are common components of the sandstones, mainly in their basal part. The following 130 m of the succession is more mud-dominated, showing overall fining-upward cycles grading from brown to light grey sandstones below to siltstones and light grey shales above; the fining-upward cycles are 5 to 10 m thick (Fig. 5e). Sandstone layers are laterally continuous, showing multiple lenticular bodies; tree trunks and plant debris are common. Common sedimentary structures in the sandstones include trough cross-bedding to low-angle lamination that grade upward to planar ripple laminations. Fine-grained rocks are dominant in this package, and the coaly shales reach a maximum thickness of 5–7 m. Up-section, 200 m thin-bedded, fine-grained sandstones, siltstones and shales with fining-upward pattern commonly contain tree trunks and plant debris. In this unit, macroflora is best preserved in siltstones. The uppermost 210 m of the measured section (from the 590 m level) is sand-dominated and characterized by a distinct increase in bed thickness and grain size of sandstones. This unit consists of brown to light grey, thick-bedded, medium-grained sandstones and laminated light grey to green, fine-grained siltstones and shales. Lenticular sandstones show sharp and erosional bases, commonly being 10–12 m wide and 2 m thick. At the top, the Hojedk Formation at the Kalshaneh section is capped by a 2 m thick, dark brown to red sandstone with iron oxide crusts and pedogenic overprint (Figs. 5c, d, 6). The unconformity is usually overlain by a thin, patchy basal conglomerate of the Parvadeh Formation, consisting of well-rounded quartz granules and pebbles, which is not developed in the measured section but preserved a few hundred metres towards the south (Fig. 5a, b).

Fig. 4. Geological map of the study area in the northern Tabas Block, simplified from the Shirgesht geological map (scale 1:100 000; Aghanabati, Reference Aghanabati1994). Location of the measured section and studied drill core (BH#8) are shown (A–B).

Fig. 5. Satellite image and field views of the Hojedk Formation at the Kalshaneh section. (a) Satellite image of the study area (Kalshaneh Coal Mine) showing lithostratigraphic boundaries between the Badamu, Hojedk and Parvadeh formations. The traverse along which the stratigraphic log (blue line) has been measured and its lateral shifts are shown. (b) The Hojedk Formation unconformably overlain along the mid-Cimmerian unconformity by the thin basal conglomerate of the Parvadeh Formation. The position of the field photo is indicated in (a). (c) The Hojedk Formation capped by reddish, Fe-oxide-rich sandstones indicating subaerial exposure associated with the mid-Cimmerian unconformity. The position of the field photo is indicated in (a). (d) Close-up view of the Fe-oxide-rich sandstone related to the mid-Cimmerian unconformity. (e) Typical fining-upward cycles grading from sandstones and siltstone to coaly shales in the middle part of the Hojedk Formation. For legend see Figure 6.
4.b. Core section BH#8
The borehole targeted for subsurface exploration of coal seams was drilled in the lowermost part of the Hojedk Formation at the Kalshaneh Coal Mine in 2017 (BH#8; 34° 10′ 56.43″ N, 56° 39′ 50.22″ E). The drill site is located ∼1.3 km to the south of the measured outcrop section (Fig. 4). The basal part of the drill core (from 167.4 m to the final depth at 187.2 m) represents the sandy bioclastic limestones of the uppermost Badamu Formation. At 167.4 m depth, the Hojedk Formation starts and includes several fining-upward cycles of light grey, medium- to fine-grained, trough cross-bedded sandstones (rarely pebbly at the base), dark grey, very fine-grained sandstones and siltstones, carbonaceous shales and coals. In total, the cored part of the lower Hojedk Formation contains seven coal seams of which only the thickest and workable seams are visible in the measured section due to the weathering.

Fig. 6. Stratigraphic log of the Hojedk Formation in the Kalshaneh section, northern Tabas Block.
4.c. Sedimentary facies and facies associations
Based on lithology, texture and sedimentary structures as well as geometry and bedding contacts of the strata, ten characteristic sedimentary facies were identified in the field. Coarse-grained facies are absent in the Hojedk Formation at Kalshaneh, while six medium-grained (St, Se, Sp, Sr, Sh, Sl), two fine-grained (Fl, Fm), one interbedded sandstone–claystone (Sr/Fl) and one organic facies (C) are recognized. Major characteristics of the facies are summarized in Table 1 and Figure 7a–l.
Table 1. Lithofacies of the Hojedk Formation at Kalshaneh section, northern Tabas Block (lithofacies code modified after Miall, Reference Miall2014)

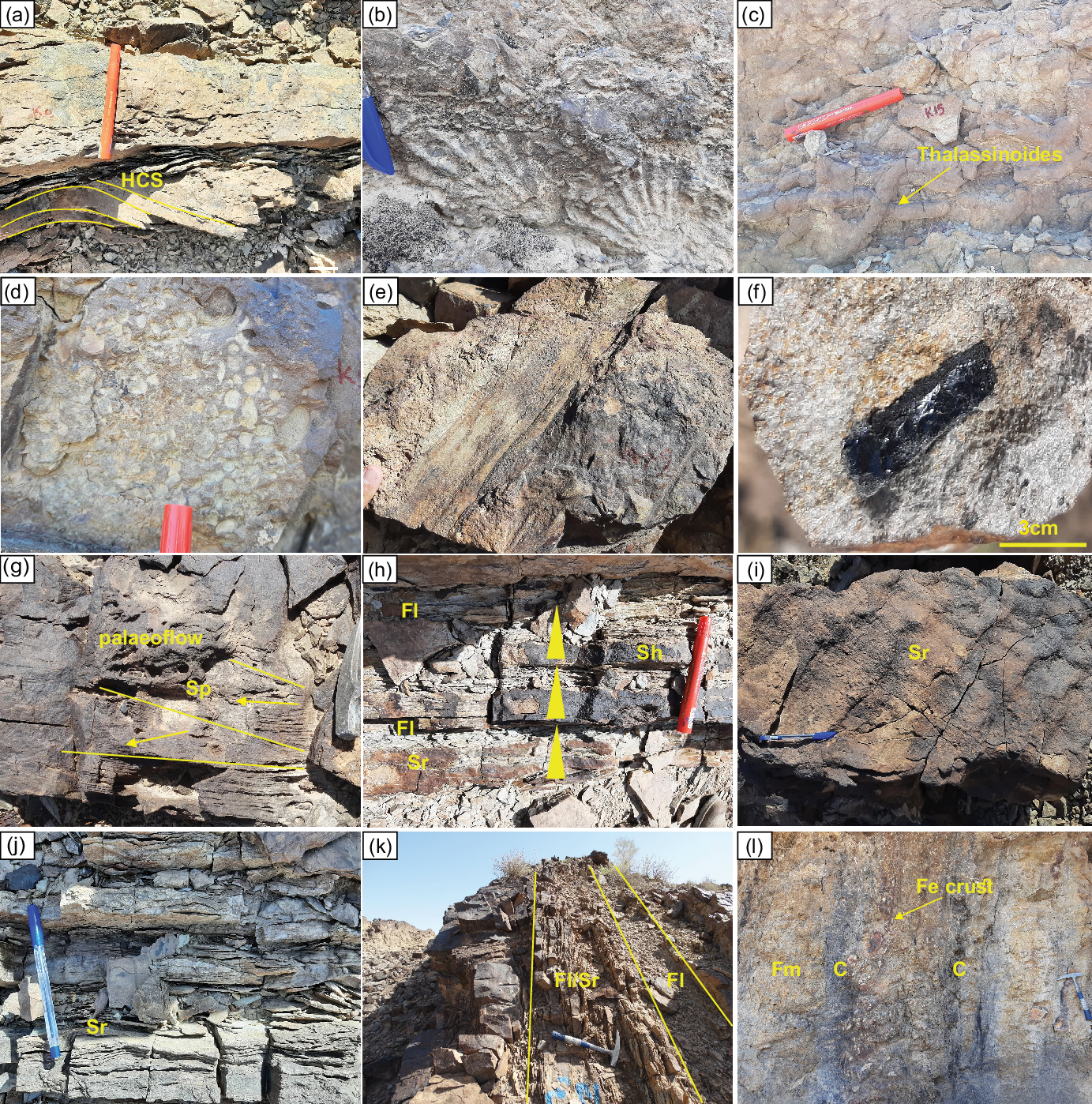
Fig. 7. Field aspects of the representative lithofacies of the Hojedk Formation in the Kalshaneh section. (a) Hummocky cross-stratified (HCS) sandstone of the shallow-marine transitional facies at the base of the section at c. 1 m. (b) Calcareous sandstone with bivalve shells (Ctenostreon sp.) from the shallow-marine transitional facies at the base of the section at c. 0 m. (c) Thalassinoides burrows in the shallow-marine sandstone at the base of the section at c. 15 m. (d) Mud chips at a basal erosional surface of a sandstone bed at c. 24 m. (e) Tree trunk and plant debris within the sandstone of a channel base from the lower part of the section at c. 79 m. (f) Medium-grained sandstones with coal clasts from a channel base of the upper part of the section at c. 615 m. (g) Cross-bedded sandstones (Sp) with unimodal palaeoflow directions from the lower part of the section at c. 166 m. (h) Rippled and planar-bedded sandstones (Sr, Sh) intercalated with laminated siltstones (Fl) from the middle part of the section at c. 362 m. (i, j) Current-rippled (Sr) surfaces on fine-grained sandstones from the lower and middle part of the section at c. 175 m and 185 m, respectively. (k) Interbedded rippled sandstones and mudstones (Fl/Sr) from the base of the section at c. 173 m. (l) Massive siltstone–claystone (Fm) grading to coal (C) from the middle part of the section at c. 353 m. Fe crusts and concretions are associated with these lithofacies.
The most common sedimentary facies within the succession is trough and planar cross-bedded sandstone (St, Sp). These facies are mostly very fine- to fine- and in places medium-grained quartz-rich, mature to super-mature sandstones. The succession most commonly consists of stacked, up to 10 m thick fining-upward cycles, which start at the base with facies Se, St and Sp and grade upwards into fine-grained facies, including ripple cross-laminated sandstone (Sr), planar laminated siltstone (Fl) and, finally, coaly shale (C) and mud rock (Fm). Coals (C) are also common in the cycles, especially in the lower part of the succession (Fig. 6).
Based on the lateral and vertical sedimentary facies distribution within the studied succession, three major facies associations (FA) have been identified that represent the main subenvironments of the overall depositional environment.
4.c.1. Facies association 1
Trough and planar cross-bedded sandstones (St, Sp) form sandstone bodies with lenticular shapes, commonly resting with abrupt grain-size increase and sharp erosional (Se) convex base on fine-grained facies and having a flat top (mostly Sh, Sr). The erosional basal boundary cuts down into fine-grained and/or organic facies of the underlying cycle. The lenticular sandstone bodies appear in the form of single, isolated beds in fine-grained facies or as laterally connected multi-storey lenses. The vertical and lateral arrangement of sedimentary facies is indicative of fluvial channel-fill deposits (Miall, Reference Miall2014).
4.c.2. Facies association 2
Planar and low-angle cross-bedded sandstones (Sp, Sl) form sigmoidal-shaped bodies with lateral accretion surfaces and change laterally and vertically to ripple laminated sandstone (Sr) and laminated, fine-grained siltstone (Fl); in places, these two facies are interbedded (Sr/Fl). The lateral accretion surfaces are curved and tangential to the lower surface of the sigmoidal-shaped sandstones. The lateral and vertical facies arrangement in the sigmoidal-shaped bodies indicates that this FA formed through lateral accretion in fluvial channels (Miall, Reference Miall2014).
4.c.3. Facies association 3
FA3 consists mainly of laminated and structureless, fine-grained facies (Fl, Fm), commonly forming tabular and sheet-like depositional units. Facies Sh and Sr are subordinate in this FA. The commonly associated coals and carbonaceous shales contain rootlet structures and ferruginous crusts. Wedge-shaped, sharp-based, fine-grained, parallel laminated sandstones (Sh), a few metres wide, are rarely interbedded within the fine-grained facies.
The sedimentological character of the drill core was used to supplement the facies data recognized in the outcrop. The representative facies in the core, with well-developed internal sedimentary structures, are illustrated in Figure 8a–i. The three principal FAs recognized in the outcrop are observed in the drill core, too. An advantage of the fresh drill core is that it better displays the delicate sedimentary structures (e.g. interlaminated bedding, ripple cross-lamination) and the thin coal seams that are absent in the surface exposures.
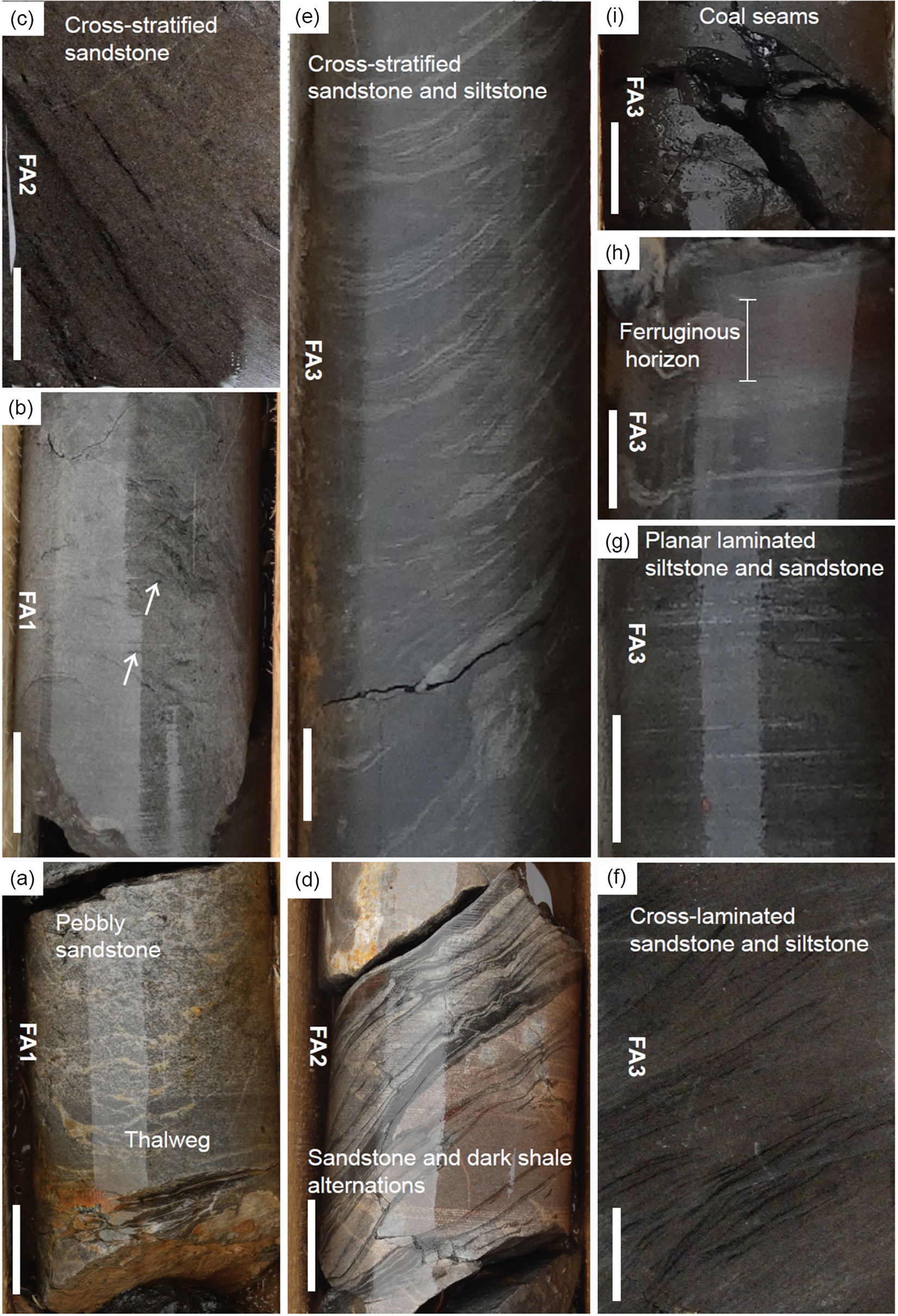
Fig. 8. Overview of representative lithofacies of the Hojedk Formation in drill core BH#8 at the Kalshaneh Coal Mine. Width of the core is 6 cm and stratigraphic-up is towards the image top. The vertical scale bar is 2 cm long in all photographs. Facies associations (FA): FA1 = channels; FA2 = point bars; FA3 = floodplains. (a) Pebbly sandstones characteristic of the thalweg of a channel at 103 m core depth. (b) Light grey, medium-grained sandstones with intraformational dark grey and black mud and coal clasts at the base (white arrows) representing FA1, grading into trough cross-bedded sandstones (FA2) at 93.1 m core depth. (c) Slabbed core segment showing medium-grained, cross-bedded sandstones interbedded with darker streaks of organic-rich mudstones of FA2 at 77.1 m core depth. (d) Interbedded dark shale and light red, medium-grained sandstones representing upper part of FA2 at 133 m core depth. (e) Dark grey, cross-bedded sandstones at 128.90 m core depth. (f) Slabbed core segment showing fine-grained sandstones with cross- to climbing ripple-lamination and siltstones of FA3 at 47.5 m core depth. (g) Dark grey, planar-laminated siltstones and fine-grained sandstones at 49 m core depth. (h) Dark grey laminated mudstones and medium grey fine-grained siltstones (FA3) with intercalated reddish-brown ferruginous horizon (possibly a palaeosol) at 134 m core depth. (i) Dark grey, structureless mudstones and coal seams at 79.30 m core depth.
4.d. Palaeocurrent analysis
The palaeocurrent data were obtained from oriented sedimentary structures, including several stacks of trough- and planar-cross-bedded foresets (32 measurements) within lenticular sandstone beds throughout the succession. The results indicate two main transport directions perpendicular to the channel axes (Fig. 6), and imply sediment supply from north and south. The implication of these transport directions is addressed in the context of the inferred depositional environment and provenance analysis below.
4.e. Petrography and modal analysis
Sandstone petrography can provide important information about the tectonic setting, source area and its exposed rock types and weathering intensity as well as the source-to-sink history of transport, deposition and diagenesis that would affect the texture and composition of the sandstones (Dickinson & Suczek, Reference Dickinson and Suczek1979; Dickinson et al. Reference Dickinson, Beard, Brakenridge, Erjavec, Ferguson, Inman, Knepp, Lindberg and Ryberg1983; Boggs, Reference Boggs2009). The grain-size analysis, based on a comparative grain-size scale in the field and on the subsequent examination of scanned petrographic thin-section images, indicates that the sandstones of the Hojedk Formation are mostly fine-grained and occasionally medium-grained; coarser-grained facies has not been observed. The sandstones are moderately well-sorted and silica-cemented (Fig. 9a, b). The main framework components comprise quartz (an average of 90 % total, which is mostly unstrained monocrystalline quartz), sedimentary and metamorphic rock fragments (on average, 8 % Lt), subordinate feldspar (on average, 2 %; typically altered potassium feldspar) and scarce, mostly resistant heavy minerals (Fig. 9c; Table 2). Stretched polycrystalline quartz grains constitute a subordinate but, as a provenance indicator, important quartz type in the sandstones (Fig. 9d). Chert fragments and, to a lesser extent, siltstone and shale clasts are common sedimentary rock fragments (Fig. 9c, d). Sand-sized coal fragments are another minor constituent in some of the samples (Fig. 9a). Some samples contain <5 % of pseudomatrix due to degradation of soft, unstable sedimentary rock fragments and/or alteration of minor feldspars grains. Hematite and Fe-rich carbonate cement is a common diagenetic feature in a few samples (Fig. 9e, f).
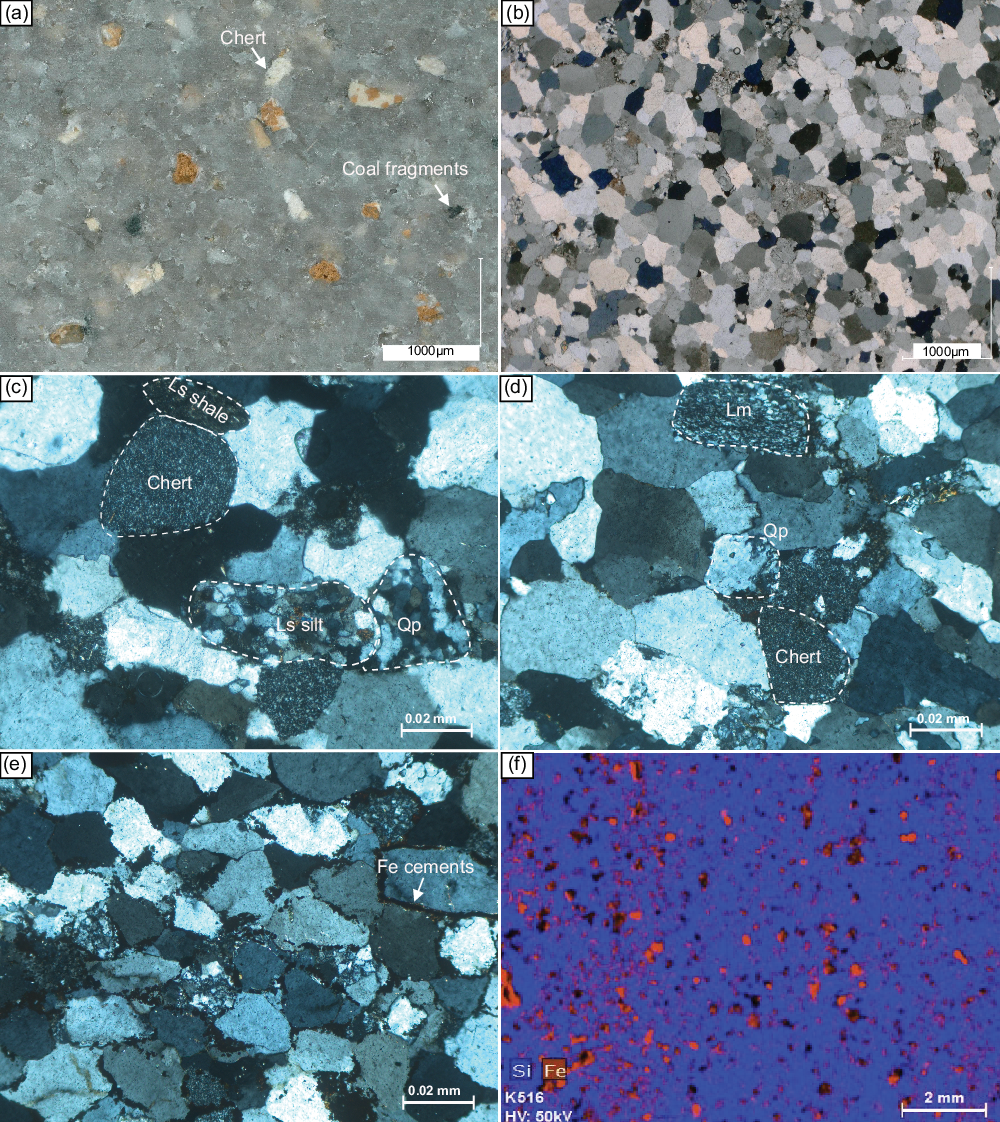
Fig. 9. Microscopic images of representative sandstone samples from the Hojedk Formation of the Kalshaneh section. (a) High-resolution photograph of a polished sandstone slab, showing well-sorted and silica-cement-rich sandstone with silt- to sand-sized coal fragments (sample K615). (b) Thin-section photomicrograph of well-sorted litho-quartzose sandstone (sample K615). (c) Polycrystalline quartz (Qp), chert, siltstone (Ls silt) and shale (Ls shale) sedimentary rock fragments (sample K666). (d) Stretched polycrystalline quartz (Qp), metamorphic (Lm) and chert rock fragments (sample K542). (e) Fe-oxide-rich carbonate cemented sandstone (sample K516). (f) μ-XRF element map of Fe-oxide-rich cemented sandstone. Si (blue) and Fe (red) represent quartz and Fe oxide cement, respectively (sample K516). For sample locations see Figure 6.
Table 2. Framework grains of 16 sandstone samples of the Hojedk Formation at Kalshaneh section, northern Tabas Block

Q = quartz; RF = rock fragments; F = feldspar.
Compositionally, most of the sandstones of the Hojedk Formation from the Kalshaneh section plot into the field of quartzarenite, while only a few samples fall into the litho-quartzose field of the newly proposed QFL plot of Garzanti (Reference Garzanti2019) or into the sublitharenite field (Folk, Reference Folk1980; Fig. 10). The latter compositional fields indicate mature quartz-rich sandstones with subordinate sedimentary and low-grade metamorphic grains.

Fig. 10. Classification of sandstone samples from the Hojedk Formation at Kalshaneh in the ternary QFL diagram of Garzanti (Reference Garzanti2019). Q = quartz, F = feldspar, L = lithic fragments. For the abbreviations of the six central fields, see Garzanti (Reference Garzanti2019).
The organic petrographic studies of representative coal samples from the main workable coal seams, stratigraphically equivalent to the lower part of the section at c. 32 m, indicate that the major macerals in the coal samples are vitrinite and inertinite (Fig. 11a–c). Clay minerals and pyrite are common associated minerals in the samples. The VRr of the coal samples ranges from 1.81 % to 2.18 %, corresponding to low-volatile bitumen to semi-anthracite (Hartkopf-Fröder et al. Reference Hartkopf-Fröder, Königshof, Littke and Schwarzbauer2015; Fig. 12).

Fig. 11. Photomicrograph images of coal samples from the Hojedk Formation at the Kalshaneh coalfield (under oil immersion with reflected light). (a) Typical vitrinite (v) and inertinite (semifusinite) (I) macerals of coal sample JHK-3. Pyrite and framboidal pyrite present in the lower right. (b) Typical vitrinite (v) and inertinite (I) macerals of coal sample JHK-1. (c) Vitrinite macerals of coal sample JHK-2. The dark laminations are clay minerals and the bright band in the centre is inertinite (I). For sample locations see Figure 6.

Fig. 12. Vitrinite reflectance distribution of the three coal samples (JHK-1–3).
4.f. Heat flow and calibration
The calibration of the model is achieved based on measured vitrinite reflectance of three coal samples from the lower Middle Jurassic Hojedk Formation. No temperature data are available for present-day thermal calibration. A semi-constant heat flow of c. 46 mW m−2 was assumed from the Silurian to the Late Triassic. From the Early Jurassic until the present day, excluding magmatic activity, a higher basal heat flow of 50 mW m−2 was assigned in the model due to the proximity of the study area to the deep-seated block-bounding Kalmard Fault that was active following the Eo-Cimmerian Event (Salehi et al. Reference Salehi, Moussavi-Harami, Mahboubi, Fürsich, Wilmsen and Heubeck2018). Magmatic activity was modelled using the PetroMod ‘intrusion tool’ and replacement of the Eocene host layer by a sill- and/or dyke-like intrusion with andesitic composition during the earliest Eocene at c. 55.86 Ma. The ‘intrusion tools’ parameters were set to an intrusion temperature of 950 °C with a magma density of 2750 kg m−3, a magma heat capacity of 0.7 kcal kg−1 K−1 and a crystallization heat of 700 MJ m−3 (e.g. Kutovaya et al. Reference Kutovaya, Kroeger, Seebeck, Back and Littke2019).
4.g. Burial, thermal and maturity history
The model comprises 12 layers ranging from the Early Ordovician to Miocene, covering a sedimentary thickness of more than 3500 m with a single erosional phase in the last 5 Ma (Table 3). According to the model results, the sedimentation in the Kalshaneh area is generally characterized by a low sedimentation rate of c. 10 m Ma−1 during the Early Ordovician to Oligocene. Exceptions are periods of rapid sedimentation during the Early Jurassic to early Middle Jurassic (65 m Ma−1), late Middle Jurassic to Late Jurassic (120 m Ma−1) and Miocene (100 m Ma−1) (Fig. 13). The sedimentation pattern controls the burial depth and therefore the temperature and maturity of different units through time. Accordingly, the Hojedk Formation was buried to c. 2300 m already by the Late Jurassic. Following low sedimentation rates during the Cretaceous to Palaeogene, the burial depth of the formation reached 3400 m in the Late Oligocene. However, rapid sedimentation in the Miocene led to a rather deep burial of up to 5000 m for this unit. The uplift and erosional event during the last 5 Ma shifted the Hojedk Formation to a shallower depth of c. 1200 m. During this time, the temperature experienced by the Hojedk Formation increased from 100 °C by the Late Jurassic to 136 and 185 °C in the Late Oligocene and Late Miocene, respectively. The late uplift in the region resulted in temperature cooling to c. 65 °C at the present day. In line with temperature variations, the maturity of the Hojedk Formation increased from 0.5 %Ro in the Late Jurassic to c. 1 %Ro in the Late Oligocene. Following the rapid sedimentation during the Miocene, the maturation reached c. 1.9 %Ro, which increased slowly up to the present day.
Table 3. Input data for maturation modelling in the Kalshaneh area, northern Tabas Block (timescale after Cohen et al. Reference Cohen, Finney, Gibbard and Fan2013)

LiSt = limestone; Ss = sandstone; Dol = dolostone; Cong = conglomerate; Gyp = gypsum; PWD = palaeowater-depth; Fm/fms = Formation/formations.

Fig. 13. Burial and thermal history in the Kalshaneh area (a), reconstructed palaeo-water depth, inferred sediment–water interface temperature (SWIT) and assumed basal heat flow through time (b) and model calibration (c).
The results indicate that the Eocene magmatic activity in the region did not directly affect the temperature and therefore the maturity of the Jurassic layers in the study region. This can be explained by the presence of more than 3000 m of sedimentary strata between the Eocene magmas and the deeply buried Jurassic strata, preventing any effects on the thermal maturation. According to the model, for a short period, the temperature reached up to more than 1000 °C in the early Eocene. This high temperature mainly affected the underlying Palaeocene strata and elevated their temperature up to 500 °C. Consequently, the maturity of the Palaeocene strata was elevated up to 3.8 %Ro. No prominent effect has been modelled for the deeper strata, e.g. Upper Cretaceous and underlying units (see discussion below).
5. Discussion
5.a. Depositional environment
Sedimentary facies analyses of the Hojedk Formation led to the identification of a thin basal shallow marine facies, only reaching 20 m in thickness, including HCS, marine shell debris and trace fossils (Fig. 6). This basal marine unit shows a gradual transition with an overall regressive trend from the underlying deeper marine, ammonite-bearing Badamu Formation (Seyed-Emami et al. Reference Seyed-Emami, Schairer, Fürsich, Wilmsen and Majidifard2000, Reference Seyed-Emami, Fürsich, Wilmsen, Schairer and Majidifard2004 a, Reference Seyed-Emami, Wilmsen and Fürsich2020). However, based on sedimentary facies analysis, most of the Hojedk Formation at Kalshaneh was deposited in a continental, fluvial depositional environment that was characterized by widespread development of channel deposits as well as thick, well-developed siltstones and coal-bearing strata. The succession is characterized by three FAs that show several classical fluvial channel fills (FA1) and laterally accreted sandstones (FA2) that are associated with fine-grained inter-channel and abandoned channel deposits, represented by coaly shales and coal horizons (FA3, Fig. 14a). The basal erosional surface of sandstone beds with associated mud chips, coal fragments and plant materials as well as pebbles in a sandy matrix indicates the thalweg of the channels (e.g. Wang & Bhattacharya, Reference Wang and Bhattacharya2017; Fig. 14b). Channels are in places connected and superimposed onto each other and form sandstone layers of fairly constant thickness within the limits of the exposure. The sandstone facies that compose the channel fills show conspicuous stratal architectures such as sigmoidal-shaped bodies internally structured by lateral accretion surfaces, providing evidence for point bar deposition and its lateral migration patterns (FA2; e.g. Fustic et al. Reference Fustic, Hubbard, Spencer, Smith, Leckie, Bennett and Larter2012; Miall, Reference Miall2014; Fig. 14c). The facies distribution and the architecture of the depositional units within the succession indicate that, occasionally, the laterally accreted deposits were cut off and a narrow channel incised into the top of the point bars forming chute channels with over-bar flows, probably during flood events (Fig. 14d). The fine-grained facies, which is mainly composed of ripple cross-laminated sandstones, planar laminated siltstones, dark grey mudrocks and shales with abundant plant material and rootlets, indicate deposition from the lower flow regime or suspension in floodplain and overbank sub-environments, while coal was formed in associated swampy floodplains (FA3; Fig. 14e). The thin-bedded wedge-shaped sandstones with a restricted lateral distribution within floodplain strata are interpreted as crevasse splays that formed during flooding events (Fig. 14f).

Fig. 14. Field aspects of the representative facies associations (FA) of the Hojedk Formation in the Kalshaneh section. (a) Channel sandstone FA (CH) with fining-upward pattern from the lower part of the section at c. 55 m. (b) Erosional channel base with pebbles and/or mud chips in a sandy matrix, forming a basal lag in the middle part of the section at c. 448 m. (c) Sigmoidal-shaped channel sandstone (CH) showing low-angle lateral accretion surfaces indicating point bar migration, intercalated between soft-weathering, fine-grained floodplain deposits (FP) from the lower part of the section at c. 228 m. (d) Chute channel cutting into a point bar deposit (PB); note floodplain deposits (FP) above (lower part of the section at c.138 m). (e) Fining-upward channel sequence grading from channelized sandstone (CH) and accreted point bar deposits (PB) to fine-grained lithofacies and coal of the floodplain FA (FP) in the middle part of the section at c. 340 m. (f) Fine-grained sandstone (CS) with limited lateral extent and wedge-shaped geometry indicating crevasse splays within the floodplain environment (middle part of the section at c. 525 m).
The core of the lowermost part of the Hojedk Formation shows several well-preserved fining-upward cycles typical of a low-energy, high-sinuosity fluvial setting. The facies in the core consists of (occasionally pebbly) medium-grained channel sandstones at the base to trough cross-bedded fine-grained sandstones and siltstones of point bars. This succession grades into dark grey to black, planar-laminated siltstones and dark carbonaceous shales to coals of floodplains and swamps above (Fig. 8a–i).
In summary, based on the sedimentary facies distribution, including the lateral and vertical facies changes, the Hojedk Formation at Kalshaneh was deposited in a low-energy meandering fluvial environment with an extensive floodplain area that was well-vegetated and locally swampy (Fig. 15). This interpretation is supported by several lines of evidence such as common fining-upward cycles, high width/depth ratio of channels, limited or weak erosion at channel bases and rather thin lag deposits, extensive laterally accreted bedding patterns in the sandstones indicating point bar migration, and the preservation of coal, which suggests well-developed and densely vegetated swampy floodplains (e.g. Ghazi & Mountney, Reference Ghazi and Mountney2009; Miall, Reference Miall2014). Correspondingly, fossil swamps have recently been reported from the Hojedk Formation of the Kerman area, southern Tabas Block (Khalilizadeh et al. Reference Khalilizadeh, Ashouri, Ghaderi and Barbacka2022). In the lower to middle parts of the succession at Kalshaneh, the fluvial channels are mostly isolated within floodplain deposits, whereas in the upper part, the channels are more stacked and coalesced, suggesting a certain change in the fluvial regime that needs explaining (see section below). The common development of densely vegetated and temporally persistent floodplains, indicated by the preservation of thick units of fine-grained facies, shows the lack of extensive erosion and reworking and, thus, prolonged equilibrium phases during the deposition of the Hojedk Formation. The palaeocurrent measurements of laterally accreted, sigmodal-shaped sandstone bodies indicate two opposite north- and south-directed sediment transport trends within point bar deposits. These, together with palaeogeographic constraints (see below), indicate a west-to-east palaeoflow direction (present-day orientation) of the high-sinuosity meandering channels on the northern Tabas Block. The source area of the Hojedk Formation had to be located on the Yazd Block in the west and will be discussed below.

Fig. 15. Sedimentary model of the Hojedk Formation in the Kalshaneh area of the northern Tabas Block (not to scale). The swampy floodplains were the sites for accumulation of plant material and the formation of coals. For key to lithologies see Figure 6.
5.b. Provenance and potential source area
Modal analysis shows that the sandstones of the Hojedk Formation at Kalshaneh comprise quartz-rich sandstones including quartz arenite and quartzo-lithic petrofacies. The modal data of these sandstones plot into the recycled orogenic field of the QmFLt and QtFL triangles for tectonic provenance (Dickinson & Suczek, Reference Dickinson and Suczek1979; Dickinson et al. Reference Dickinson, Beard, Brakenridge, Erjavec, Ferguson, Inman, Knepp, Lindberg and Ryberg1983; Fig. 16). This supports derivation of the sediment from a recycled-orogen provenance. Very few samples plot into the craton interior provenance which indicates derivation from relatively low-lying granitoid and gneissic source rocks (Dickinson et al. Reference Dickinson, Beard, Brakenridge, Erjavec, Ferguson, Inman, Knepp, Lindberg and Ryberg1983). However, using only detrital modes for reconstructing the plate-tectonic setting is the main point of criticism by Garzanti (Reference Garzanti2016) who recommends combining sandstone petrography with multiple techniques to infer the tectono-stratigraphic conditions of source areas. The high textural maturity of the sandstone samples also supports recycling rather than first-cycle sediments. However, recycling is a physical process and cannot solely produce mature sandstones (Garzanti, Reference Garzanti2017), though strong chemical weathering under humid Middle Jurassic climate, coupled with sedimentary recycling, definitely increased the maturity of the sandstones of the Hojedk Formation. The presence of (pseudo-)matrix in some of the sandstone samples is most probably due to squeezing of mud intraclasts eroded from floodplain deposits between rigid grains (e.g. Morad et al. Reference Morad, Al-Ramadan, Ketzer and De Ros2010). Palaeogeographic constraints (exposure of the Yazd Block as indicated by the widespread absence of Middle Jurassic strata), the distribution of facies associations and palaeocurrent data from the Hojedk Formation at the Kalshaneh suggest that one of the possible source areas for the Hojedk Basin in the northern Tabas Block is represented by the exposed rocks on the Yazd Block in the west. The source rock composition of the Yazd Block is rather diverse due to the existence of several basement rock units, including Precambrian and Ordovician–Triassic sedimentary, low- to upper-rank metamorphic as well as plutonic rocks (Bagheri & Stampfli, Reference Bagheri and Stampfli2008). The Posht-e-Badam complex of the eastern Yazd Block (Kargaranbafghi et al. Reference Kargaranbafghi, Neubauer, Genser, Faghih and Kusky2012, Reference Kargaranbafghi, Neubauer and Genser2015) had been considered as a potential source for the Ab-e-Haji Formation of the western part of the Tabas Block during the Early Jurassic (Salehi et al. Reference Salehi, Moussavi-Harami, Mahboubi, Wilmsen and Heubeck2014, Reference Salehi, Moussavi-Harami, Mahboubi, Fürsich, Wilmsen and Heubeck2018). However, based on the petrographic analysis, the sandstones of the Hojedk Formation are lithic-poor, though the presence of subordinate low-grade metamorphic rock fragments and stretched polycrystalline quartz could be evidence for a source area from the Yazd Block in the west. The quartz-rich sandstones of the Hojedk Formation might originate from erosion and recycling of older quartz-rich sediments or supracrustal sedimentary rocks of the Yazd Block, while erosion probably did not unroof the underlying plutonic and metamorphic basement rocks. The silt- to sand-sized organic matter (reworked coal fragments) in the sandstone samples also indicates erosion of coal-bearing strata within the Hojedk Basin or in the source area. Potential source rocks may be the siliciclastic, coal-bearing units in the lower Shemshak Group (Upper Triassic to ?Lower Jurassic) that are present in some parts of the Yazd Block (Wilmsen et al. Reference Wilmsen, Fürsich and Majidifard2015). Furthermore, a high degree of chemical weathering in the source area due to overall very humid climatic conditions during the deposition of the Hojedk Formation is evident by the presence of rich macrofloras (e.g. Vaez-Javadi, Reference Vaez-Javadi2016) and coals as well as the prevalence of grey colours and the lack of red beds (e.g. Sheldon, Reference Sheldon and Gornitz2009). The fossil-plant-rich floodplain deposits of the Hojedk Formation in the Kalshaneh area contain macrofloras (e.g. Nilssonia sp., Ginkgoales sp.; Vaez-Javadi, Reference Vaez-Javadi2016) typical of a humid subtropical to temperate climate, and Khalilizadeh et al. (Reference Khalilizadeh, Ashouri, Ghaderi and Barbacka2022) documented extensive swamps for the Hojedk Formation of the Kerman area. Such conditions might have removed unstable lithic grains, thus increasing the quartz content and maturity of sandstones (e.g. Weibel et al. Reference Weibel, Olivarius, Kjøller, Kristensen, Hjuler, Friis, Pedersen, Boyce, Andersen, Kamla, Boldreel, Mathiesen and Nielsen2017). Modal analysis of sandstones indicates a similar quartzose recycled provenance from a metamorphic source area with humid climatic conditions for the top part of the Hojedk Formation in the southern Tabas Block (Zamani-Pedram, Reference Zamani-Pedram2011). Likewise, this study considers the exposed rocks on the Yazd Block and Kalmard sub-block to the west as the source area for the clastic sediments deposited in the southern Tabas Block.

Fig. 16. QmFLt and QtFL ternary plots (Dickinson & Suczek, Reference Dickinson and Suczek1979; Dickinson et al. Reference Dickinson, Beard, Brakenridge, Erjavec, Ferguson, Inman, Knepp, Lindberg and Ryberg1983) for tectonic provenance reconstruction of the Hojedk Formation in the northern Tabas Block. Qt = total quartz; Qm = monocrystalline quartz; F = feldspar; Lt = total lithic fragments including polycrystalline quartz grains and chert fragments; L = unstable lithic fragments.
5.c. Thermal and maturation history
The VRr measurements indicate advanced thermal maturity of the wet to dry gas zone for the analysed coal samples (Fig. 12). The maturity is comparable to that of Middle Jurassic coals from the adjacent Mazino coalfield to the south ranging from 2.08 % to 2.29 % (Zamansani et al. Reference Zamansani, Rajabzadeh, Littke, Zieger and Baniasad2019) and is far higher than the reported reflectance for the Upper Triassic coals in the Parvadeh coalfield ranging from 1.19 % to 1.33 %, corresponding to the upper oil window (see Fig. 1b for locations). In a recent study by Zamansani et al. (Reference Zamansani, Rajabzadeh, Littke, Zieger and Baniasad2019), the advanced maturation for the Jurassic coal in the Mazino area is attributed to a post-Jurassic overburden of 4500 to 6000 m according to applied basal heat flow. In the same study, a magmatic effect at 34 Ma and thick sediment deposition due to the Alpine orogeny at 20 Ma has been taken into account. However, the post-Jurassic strata on the Tabas Block are expected to be far thinner (e.g. Huckriede et al. Reference Huckriede, Ku Rsten and Venzlaff1962; Wilmsen et al. Reference Wilmsen, Wiese, Seyed-Emami and Fürsich2005; Zamani-Pedram, Reference Zamani-Pedram2011). All in all, the thickness of the strata overlying the Shemshak Group prior to erosion did not exceed c. 4350 m (Table 3).
After examining different scenarios in the model, the best calibration with the new kinetic model of Easy %RoDL (Burnham, Reference Burnham2016) was achieved by considering a relatively deep burial depth which is consistent with the thickness of the lithostratigraphic units overlying the Shemshak Group in the type or reference sections on the northern Tabas Block before erosion. These strata include the upper Middle Jurassic to Palaeocene sedimentary succession, Eocene–Oligocene volcanic rocks, thick (c. 1500 m) Neogene red beds and conglomerates, equivalent to the Upper Red Formation, causing a maximum burial depth during the Miocene (Table 3; Fig. 13). In order to reach the calibration, a minimum of 3700 m of erosion is necessary in the model, which is in agreement with the present-day thickness of the overburden after a major uplift and erosion event during the Alpine orogeny sensu lato from 34 Ma to 0 Ma (Agard et al. Reference Agard, Omrani, Jolivet, Whitechurch, Vrielynck, Spakman, Monié, Meyer and Wortel2011); most of the exhumation in the northern CEIM occurred rather late from 5 Ma to 0 Ma (Konon et al. Reference Konon, Nadimi, Koprianiuk, Wysocka, Szaniawski, Wyglądała, Słaby, Beygi and Barski2016; Tadayon et al. Reference Tadayon, Rossetti, Zattin, Nozaem, Calzolari, Madanipour and Salvini2017) (Table 3).
In addition to the attributed maximum burial depth, the present-day thermal state of the coals from the Hojedk Formation at Kalshaneh may result from an advanced thermal maturation of the region caused by, for example, hydrothermal activities along the adjacent deep-seated block-bounding faults and/or intense magmatic activity during the Palaeogene (e.g. Kargaranbafghi et al. Reference Kargaranbafghi, Neubauer and Genser2015; Mehdipour Ghazi et al. Reference Mehdipour Ghazi, Moazzen, Rahgoshay and Wilde2020). The latter scenario is further supported by evidence of a regional magmatic event during the Eocene in the northern Tabas Block (Stöcklin et al. Reference Stöcklin, Eftekhar Nezhad and Hushmand-Zadeh1965; Ruttner et al. Reference Ruttner, Nabavi and Hajian1968; Konon et al. Reference Konon, Nadimi, Koprianiuk, Wysocka, Szaniawski, Wyglądała, Słaby, Beygi and Barski2016) and on the northern Yazd Block in the Khur area (Aistov et al. Reference Aistov, Melnikov, Krivyakin, Morozov and Kiristaev1984). Although the modelling did not show a direct effect of magmatic activity on the thermal maturity of the Middle Jurassic coals, the vicinity of the study area to the deep-seated Kalmard Fault (see Figs 1, 4 for location) implies a mixed scenario affecting the thermal maturation of the region by means of a slightly increased heat flow since the Early Jurassic when the fault became active (Salehi et al. Reference Salehi, Moussavi-Harami, Mahboubi, Fürsich, Wilmsen and Heubeck2018). There is also a possibility that the maturity of Middle Jurassic coal-bearing strata was affected by possible Eocene magmas that were emplaced in the subsurface and intruded the area in the form of sills or dykes. Although there are few documented occurrences of Eocene magmatic rocks in the northern Tabas Block, the factual record of volcanic or sub-volcanic rocks in the study area is sparse due to the later erosion. However, on a slightly larger regional scale there is widespread field evidence of Eocene to Oligocene sub-volcanic rocks with tens of metres-thick sills intruding Lower Cretaceous sedimentary strata in the Khur area of the northern Yazd Block (Aistov et al. Reference Aistov, Melnikov, Krivyakin, Morozov and Kiristaev1984, and unpublished data from the Rudkhaneh–Arusan area, south of Chupanan). Therefore, we cannot rule out a similar geological situation for the Kalshaneh area with sub-volcanic intrusions penetrating post-Shemshak strata and magnifying the thermal maturation of the coals within the Hojedk Formation. A detailed regional mapping survey would be needed in order to better understand the effects of Eocene to Oligocene magmatic activity on the thermal maturation of the Jurassic coals on the northern Tabas Bock. However, such investigation is beyond the scope of the present study.
5.d. Palaeogeographic implications
In this study, the stratigraphy and sedimentary facies analysis of the studied section, including palaeocurrent measurements and petrographic analysis of sandstones, are considered in order to reconstruct the palaeogeographic framework of the Hojedk Formation during the Middle Jurassic and the geological processes prior to the Mid-Cimmerian (Bajocian) tectonic event.
After the widespread transgression during the Toarcian–Aalenian, which resulted in the formation of the ammonite-rich limestones of the Badamu Formation in large parts of the CEIM (e.g. Seyed-Emami et al. Reference Seyed-Emami, Fürsich, Wilmsen, Schairer and Majidifard2004 a) and the deposition of deep-marine facies also in other parts of Iran (e.g. Fürsich et al. Reference Fürsich, Wilmsen, Seyed-Emami, Cecca and Majidifard2005 b), the accumulation of the Hojedk Formation during the early Bajocian indicates rapid shallowing and progradation of facies belts. Thickness and facies variations over short distances have been reported as well (Wilmsen et al. Reference Wilmsen, Fürsich and Seyed-Emami2003, Reference Wilmsen, Fürsich, Seyed-Emami, Majidifard, Brunet, Wilmsen and Granath2009 b). This points to tectonic instability in the run-up to the Mid-Cimmerian tectonic event (Fürsich et al. Reference Fürsich, Wilmsen, Seyed-Emami, Majidifard, Brunet, Wilmsen and Granath2009 a; Wilmsen et al. Reference Wilmsen, Fürsich, Seyed-Emami, Majidifard, Brunet, Wilmsen and Granath2009 b). Based on the geodynamic models presented for the CEIM from the Early to early Late Jurassic (Wilmsen et al. Reference Wilmsen, Fürsich, Seyed-Emami, Majidifard and Taheri2009 a, Reference Wilmsen, Fürsich, Seyed-Emami and Majidifard2021; Mattei et al. Reference Mattei, Cifelli, Muttoni and Rashid2015), the three blocks of the CEIM (Yazd, Tabas and Lut) were in a pre-rotational position, their long axes approximately oriented W–E and the Lut Block facing the Neotethys in the south. Their bounding faults acted as normal faults, which explains the lithostratigraphic variation and facies patterns of the siliciclastic-dominated Shemshak Group and the carbonate-, mixed carbonate/clastic- and evaporite-dominated Magu Group Wilmsen et al. Reference Wilmsen, Fürsich, Seyed-Emami, Majidifard, Brunet, Wilmsen and Granath2009 b, Reference Wilmsen, Fürsich, Seyed-Emami, Majidifard and Zamani-Pedram2010; Salehi et al. Reference Salehi, Moussavi-Harami, Mahboubi, Wilmsen and Heubeck2014, Reference Salehi, Moussavi-Harami, Mahboubi, Fürsich, Wilmsen and Heubeck2018; Fürsich et al. Reference Fürsich, Pan, Wilmsen and Majidifard2016; Seyed-Emami et al. Reference Seyed-Emami, Wilmsen and Fürsich2020). The extensional tectonic regime occurred in a continental retro-arc basin during phases of limited frictional stress in the subduction zone of the Neotethys Ocean to the south, the crust of which was subducted beneath the Iran Plate (Wilmsen et al. Reference Wilmsen, Fürsich, Seyed-Emami, Majidifard and Taheri2009 a; Salehi et al. Reference Salehi, Moussavi-Harami, Mahboubi, Fürsich, Wilmsen and Heubeck2018). However, several short-term tectonic events punctuated the Jurassic succession of the Tabas Block (Fürsich et al. Reference Fürsich, Wilmsen, Seyed-Emami and Majidifard2003; Wilmsen et al. Reference Wilmsen, Fürsich and Seyed-Emami2003, Reference Wilmsen, Fürsich, Seyed-Emami, Majidifard, Brunet, Wilmsen and Granath2009 b, Reference Wilmsen, Fürsich, Seyed-Emami and Majidifard2021; Seyed-Emami et al. Reference Seyed-Emami, Fürsich and Wilmsen2004 b) that may have had their origin in subduction-related processes to the south of the CEIM (e.g. sudden changes in subduction rate and/or variations of frictional stress).
The replacement of marine carbonates of the Badamu Formation by the siliciclastic rocks of the Hojedk Formation was probably caused by uplift of the Yazd Block with extensive erosion that supplied large amounts of clastic sediments to the Hojedk Basin (Fig. 17). The great thickness (800 m) of fluvial strata of the Hojedk Formation at Kalshaneh points to a pronounced extension-related subsidence in the sedimentary basin of the northern Tabas Block. The rapid rate of differential subsidence could be explained by accelerated normal block-faulting in the back-arc extensional basin of the CEIM and contemporaneous N–S extension in northern Iran and the South Caspian Basin (Brunet et al. Reference Brunet, Korotaev, Ershov and Nikishin2003; Fürsich et al. Reference Fürsich, Wilmsen, Seyed-Emami, Cecca and Majidifard2005 b, Reference Wilmsen, Fürsich, Seyed-Emami, Majidifard and Taheri2009 a; Wilmsen et al. Reference Wilmsen, Fürsich, Seyed-Emami, Majidifard and Taheri2009 a). This presence of two parallel zones of extension will form a domain of compression in between, explaining the uplift of the northern basin shoulder (Yazd Block) of the CEIM. However, the fault-bounded, rapidly subsiding Tabas Basin was rapidly filled with clastic sediments of a low-energy and low-relief meandering river system flowing from the basin margin in the north (Yazd Block) southward (Lut Block; in present-day block orientation W–E) (Fig. 17). This fluvial environment prevailed on the western Tabas Block (Kalmard sub-block) and was replaced by marginal and shallow marine environments toward to the east, evidenced by marine facies of the Hojedk Formation on the eastern Tabas and Lut blocks (Seyed-Emami et al. Reference Seyed-Emami, Fürsich, Wilmsen, Schairer and Majidifard2004 a; Wilmsen et al. Reference Wilmsen, Fürsich, Seyed-Emami, Majidifard, Brunet, Wilmsen and Granath2009 b). The presence of meandering-rivers sedimentary facies within the succession confirms the deposition in an extensional and relatively low-gradient area rather than compressional setting for the (northern part of the) Tabas Block. Moreover, the uniform stacking of fluvial fining-upward cycles in large parts of the succession indicates continuous basin subsidence and uplift of the source area during an equilibrium phase of differential subsidence. The pronounced increase in bed thickness and sandstone grain size in the upper part of the Hojedk Formation is probably a response of the sedimentary system to the Mid-Cimmerian (mid-Bajocian) compressional tectonic event and associated deformation and increased uplift in the source area (Fürsich et al. Reference Fürsich, Wilmsen, Seyed-Emami, Majidifard, Brunet, Wilmsen and Granath2009 a). This tectonic event temporarily decreased the rate of basin subsidence and resulted in the deposition of the sand-dominated succession towards the top of the Hojedk Formation. The compressional regime finally terminated the sedimentary megacycle of the Shemshak Group and led to subaerial exposure and erosion (formation of iron oxide crust, pedogenic overprint) at the top of the Hojedk Formation in the Kalshaneh area. The overlying limestones of the Upper Bajocian Parvadeh Formation with its basal quartz conglomerate indicate a renewed phase of subsidence and generation of accommodation space on the Tabas Block, which resulted in a pronounced relative sea-level rise (deposition of the Parvadeh and Baghamshah formations) and the establishment of the large-scale carbonate platform system of the Esfandiar Subgroup (Wilmsen et al. Reference Wilmsen, Fürsich and Seyed-Emami2003, Reference Wilmsen, Fürsich, Seyed-Emami, Majidifard, Brunet, Wilmsen and Granath2009 b, Reference Wilmsen, Fürsich, Seyed-Emami, Majidifard and Zamani-Pedram2010). Similar marine transgressions following a mid Bajocian (i.e. Mid-Cimmerian) uplift event occurred during the Late Bajocian in other parts of Iran such as in the Alborz, Binalud and Kopet-Dagh basins (e.g. Fürsich et al. Reference Fürsich, Wilmsen, Seyed-Emami, Majidifard, Brunet, Wilmsen and Granath2009 a; Taheri et al. Reference Taheri, Fürsich, Wilmsen, Brunet, Wilmsen and Granath2009) as well as the neighboring Afghan–Tajik Basin, which suggests a largely synchronous tectonic activity (Fürsich et al. Reference Fürsich, Brunet, Auxiètre, Munsch, Brunet, McCann and Sobel2017). Furthermore, in situ Rb/Sr dating recently yielded precise and accurate cooling ages ranging from 170 to 140 Ma that document the exhumation of Central Iran from the Mid-Cimmerian Event onward (Gyomlai et al. Reference Gyomlai, Agard, Jolivet, Larvet, Bonnet, Omrani, Larson, Caron and Noël2022) and provided a temporal link to the succeeding Late Cimmerian tectonics (Wilmsen et al. Reference Wilmsen, Fürsich, Seyed-Emami and Majidifard2021).

Fig. 17. Palaeogeography of the northern Tabas Block in east-central Iran during the Middle Jurassic (early Bajocian). The meandering river system on the Kalmard sub-block and western part of the northern Tabas Block was sourced from the Yazd Block, grading into shallow-marine lithofacies eastwards. NF: Naini Fault; KF: Kalmard Fault.
6. Conclusion
Combined outcrop and subsurface sedimentary facies analysis of the lower Bajocian Hojedk Formation in the Kalshaneh area of the northern Tabas Block resulted in the recognition of ten characteristic medium- to fine-grained facies, which were grouped into three facies associations, representing high-sinuosity meandering channels with laterally accreting point bars as well as densely vegetated swampy floodplain. Based on palaeobotanical evidence, this low-energy fluvial system formed under humid subtropical to warm-temperate climatic conditions. Based on palaeocurrent analyses, the palaeoflow in the channels was from west to east, corresponding to a north-to-south transport when considering the post-Jurassic anticlockwise vertical-axis rotation of the CEIM.
Modal analysis indicates that the mature quartz arenites and quartzo-lithic sandstones of the Hojedk Formation were derived from a recycled-orogen provenance. The strata originated by erosion and recycling of older quartz-rich sediments or supracrustal sedimentary rocks from the Yazd Block in the west, and the warm, humid Middle Jurassic climate contributed to the advanced maturation of the sediments. However, an accurate tectono-stratigraphic reconstruction of the source area will require further studies through integrated techniques.
Coal petrography analysis indicates vitrinite as a major maceral with a vitrinite reflectance (VRr) ranging from 1.81 % to 2.18 %, pointing to an advanced maturation stage (semi-anthracite). Due to the rather thin post-depositional overburden on the northern Tabas Block, a higher geothermal gradient in this tectonically highly mobile area and/or heating by Palaeogene volcanics most probably contributed to thermal maturation of the Hojedk coals.
The great thickness of fluvial strata of the Hojedk Formation at Kalshaneh points to a pronounced extension-related subsidence in the sedimentary basin of the northern Tabas Block. The rapid rate of differential subsidence can be explained by accelerated normal block-faulting in the back-arc extensional basin of the CEIM in the run-up to the Mid-Cimmerian tectonic event. The fault-bounded, rapidly subsiding Tabas Basin, including the Kalmard sub-block, was rapidly filled with clastic sediments of low-energy and low-relief meandering river systems flowing from the basin margin in the north (Yazd Block) to the south (Lut Block; in present-day block orientation W–E).
Acknowledgements
This research was supported by the University of Isfahan. We would like to thank the Tabas Geopark for support during field trips and the Kalshaneh Coal Mine for allowing access to the outcrop as well as to cores, stored in the repository at Hudar Village. We also would like to thank Mr Ahmadzadeh and Mr Ghanbari (both in Tabas) for assistance in the field. M.W. acknowledges the long-term support by the Geological Survey of Iran during field work in the country. A DAAD grant to A.S. for a research visit to the Department of Geosciences, Friedrich Schiller University Jena, Germany, is highly appreciated. The FSU Jena and the RWTH Aachen University are thanked for providing analytical support. Constructive reviews by FT Fürsich (Erlangen) and an anonymous reviewer are gratefully acknowledged. Comments and a careful revision by executive editor Emese M. Bordy (Cape Town) significantly improved the manuscript.