Introduction
In thoracic radiotherapy (RT), heart sparing is very essential, as the high cardiac dose is associated with poor survival in patients with locally advanced non-small cell lung cancer (NSCLC). One of the lead studies, the RTOG 0617 study, reported worse survival in the high-dose (74 Gy) randomised arm than the standard 60 Gy arm (median survival 20 versus 28 months). This outcome was defined as associated with elevated heart V5 and V30. Reference Bradley, Paulus and Komaki1 In addition to this study, many studies have investigated the effect of cardiac doses on survival or the incidence of cardiac events after thoracic RT. Reference Dess, Sun and Matuszak2–Reference Ma, Sun and Sun8 In a study of 112 patients with NCSLC who underwent RT, mean heart dose was found to be independently associated with cardiac events. When the mean heart dose was <10 Gy, between 10–20 Gy and >20 Gy, cardiac event rates were 4%, 7% and 21%, respectively. Reference Wang, Eblan and Deal9
In the era of positive results of immunotherapy and targeted therapies, the fate of locally advanced lung cancer has changed and treatment outcomes considerably have improved. While disease-related outcomes improve as a result of treatment, cardiac mortality is more crucial. Reference Dess, Sun and Matuszak2–Reference Wang, Eblan and Deal9 However, analyses in the literature are limited in identifying dose-related toxicity, especially of heart substructures, and toxicity-limiting dosimetric information is lacking. Cardiotoxicity can negatively affect survival outcomes, especially when mediastinal irradiation is required. While simulation with four-dimensional computed tomography (CT) is necessary for the minimum margin due to organ movement in lung cancer patients, this opportunity is not available in some clinics in more rural areas like ours, and three-dimensional conformal treatments are performed in some lung cancer patients due to the above-mentioned lack of simulation and absence of image-guided radiotherapy. For this reason, in this study, we compared conformal and static intensity-modulated radiotherapy (s-IMRT) and dynamic intensity-modulated radiotherapy (d-IMRT) plans. In this dosimetric study, we aimed to determine the doses exposed to heart substructures and coronary arteries by different RT techniques in patients with lung cancer when mediastinal irradiation is performed or not.
Material and methods
Twenty patients with NSCLC, irradiated between January 2018 and December 2020 in the Radiation Oncology clinic of Tokat Gaziosmanpaşa University, were included in this study. Patients whose primary tumour was centrally located in the left lung were selected. The central tumour was defined as follows: the proximal tracheobronchial tree (PTA) was determined according to the RTOG guideline and it was expressed as the tumour in the area formed by expanding a 3-cm margin to PTA. Patients with any T or any N located centrally in the left lung were included, whereas patients with peripheral and right-sided tumours were excluded from the study.
In addition to the target volume and critical organs, the heart chambers [left atrium (LA), right atrium (RA), left ventricle (LV) and right ventricle (RV)] and coronary arteries [left main (LMCA), left anterior descending (LAD), circumflex (CX) and right coronary arteries (RCAs)] were delineated according to contouring atlas by the Danish Multidisciplinary Cancer Groups Reference Milo, Offersen and Bechmann10 by the same physician (Figure 1). RT was planned with three different techniques for each patient using Clinac DHX linear accelerator units (the Eclipse 15.1 version treatment planning system) developed by Varian Medical System (Palo Alto, CA, USA). The doses of 60 Gy external RT were prescribed in 30 fractions using three-dimensional conformal radiotherapy (3D-CRT), s-IMRT and d-IMRT techniques in all patients (Figure 2).

Figure 1. Delineation of heart substructures-coronary arteries and their relationship with PTV in a sample case. Left atrium: brown, right atrium: blue, left ventricle: cyan, whole heart: green, left anterior descending (LAD): yellow, circumflex artery (CX): pink, planning target volume (PTV): red.
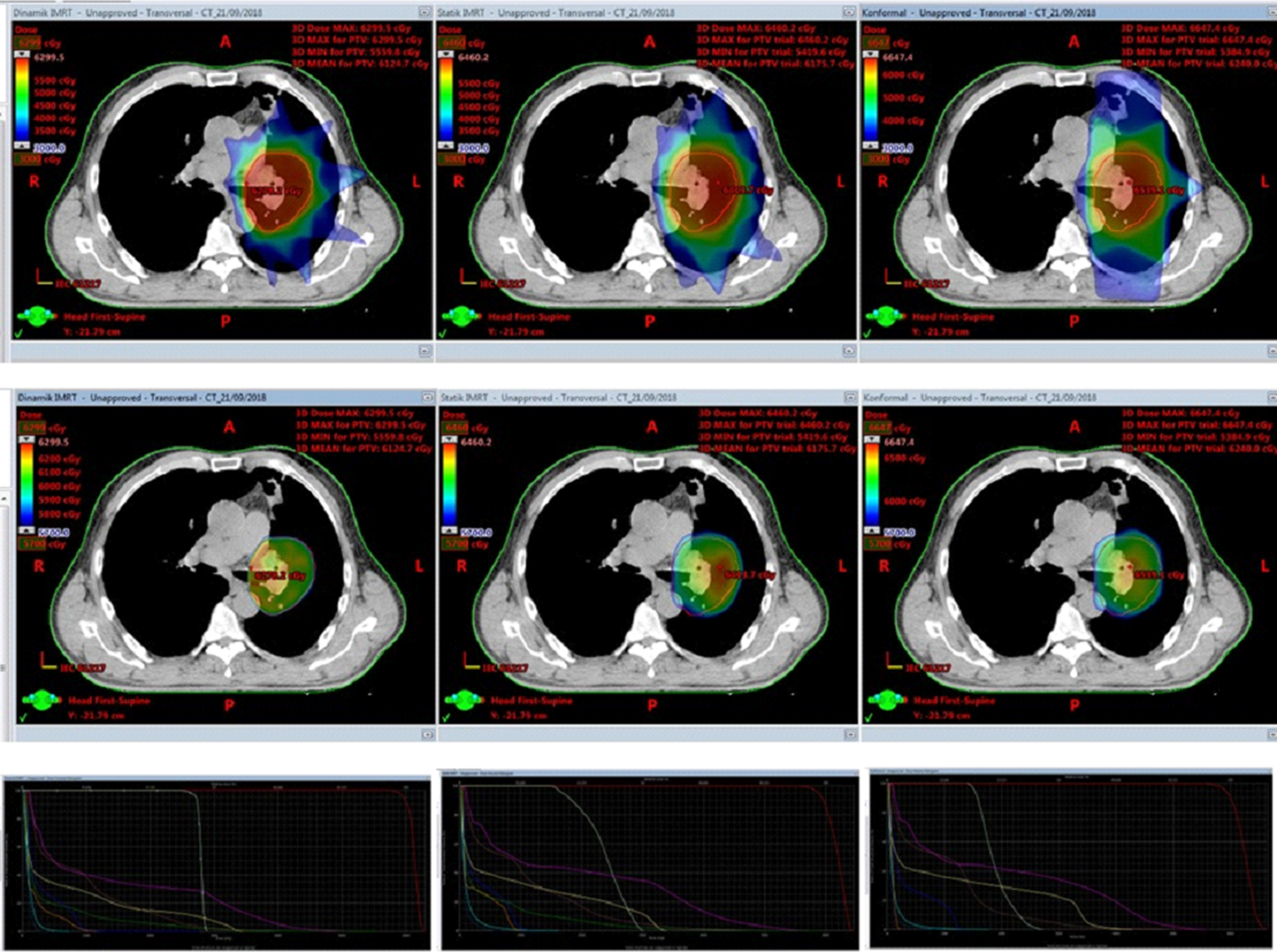
Figure 2. The plans, isodose curves and dose-volume histograms (DVH) of the sample patient with three radiotherapy techniques. From top to bottom, first column: isodose 50% (volume absorbed by 50% of dose), second column: isodose 95% (volume absorbed by 95% of dose), last column: dose-volume histograms – from left to right in order, dynamic-IMRT, static-IMRT and finally 3D conformal RT plans.
The 3D-CRT plans were made using 15 MV photon energy with fields given from four different angles. In the s-IMRT (field-in-field) plans, 6–15 MV photon energy and fixed multi-leaf collimators (MLCs) were used in four or six fields. In the d-IMRT plans, 6 MV photon energy and mobile MLCs were employed in five or six fields. Since the target volumes were located in the left lung in all plans, the fields were created from the left side.
The following parameters were evaluated in RT plans with three different techniques: Dmean (mean dose), Dmax (maximum dose),V30 (the percentage of volume receiving 30 Gy),V45 (the percentage of volume receiving 45 Gy) for the whole heart, LA-RA and ventricles; Dmean, Dmax for LMCA, LAD, CX and RCA; and Dmean, Dmax, V95 (the percentage of volume receiving 95% of the prescribed dose),V107 (the percentage of volume receiving 107% of the prescribed dose), conformity index (CI) and homogeneity index (HI) for planning target volume (PTV). For HI [zero is ideal; (D2%–D98%)/D50%] and for CI (1·0 is ideal; volume of PTV covered by the 95% isodose curve/volume of PTV) formulas were used. 11 Patients were divided into subgroups as N (node) positive (n = 11) and N negative (n = 9). The dose-volume differences of the heart substructures in three different techniques were examined according to whether or not mediastinal irradiation was performed.
Statistical analysis
The SPSS version 20 was used for statistical analysis. The doses of the heart substructures in 60 plans performed on 20 patients with 3 techniques were initially evaluated by Friedman test. Post hoc analyses were performed with the Wilcoxon test insignificant ones, and Bonferroni correction was used. Statistical significance was accepted as less than 0·05.
Results
For the whole heart
While the d-IMRT plans were statistically the best optimal plan, there was no difference between s-IMRT and 3D-CRT plans for Dmax [65 Gy (3D-CRT)-64 6 Gy (s-IMRT)- 62 6 Gy (d-IMRT), p < 0 0001], Dmean [17,5 Gy (3D-CRT)- 16 7 Gy (s-IMRT)- 13 5 Gy (d-IMRT), p < 0 0001] and V45 [10·4% (3D-conf)- 9 3% (s-IMRT)- 4 3% (d-IMRT), p < 0 0001]. For whole heart V30, the best optimal plans were d-IMRT, whereas s-IMRT was statistically superior to the 3D-CRT plans [27% (3D-conf)- 22% (s-IMRT)- 15% (d-IMRT), p = 0 009] (Table 1).
Table 1. Dose parameters to PTV and organs at risk for all 20 patients with 3D-CRT, static-IMRT and dynamic-IMRT plans. Dosimetric parameters are presented as mean values ± standard deviation (SD)

Abbreviations: PTV, planning target volume; HI, homogeneity index; CI, conformity index; Dmean, mean dose; Dmax, maximum dose; V30, the percentage of volume receiving 30 Gy; V45, the percentage of volume receiving 45 Gy; V95, the percentage of volume receiving 95% of the prescribed dose; V107, the percentage of volume receiving 107% of the prescribed dose; 3D-CRT, three-dimensional conformal radiotherapy; IMRT, intensity-modulated radiotherapy.
For PTV
The d-IMRT plans were statistically the best optimal plan for Dmean, Dmax, V95, V107, CI and HI, while all these parameters were found to be statistically significantly superior to 3D-CRT plans in the s-IMRT plans. Dmean [62 4 Gy (3D-CRT)- 62 1 Gy (s-IMRT)- 61 Gy (d-IMRT), p = 0 04], Dmax [66 7 Gy (3D-CRT)- 65 6 Gy (s-IMRT)- 63 3 Gy (d-IMRT), p < 0 0001], V95 [98 7% (3D-CRT)- 99% (s-IMRT)- 99 9% (d-IMRT), p < 0 0001] ve V107 [21 7% (3D-CRT)- 11% (s-IMRT)- 0% (d-IMRT), p < 0 0001] and CI [1 2 (3D-CRT)- 1 13 (s-IMRT)- 0 94 (d-IMRT), p < 0 0001] ve HI [0 14 (3D-CRT)- 0 11 (s-IMRT)- 0 05 (d-IMRT), p < 0 0001].
For the heart substructures
There were no differences between the three RT techniques for RA Dmax [36 6 Gy (3D-CRT)- 33 4 Gy (s-IMRT)- 30 7 Gy (d-IMRT), p = 0 148] and V45 [% 0 in all plans, p = 0 12], whereas the d-IMRT technique was significantly better for all other heart substructures (Dmax, Dmean, V30, V45 for LA, LV and RV) (the details are shown in Table 1).
For the coronary arteries
Of the coronary arteries, LMCA, LAD, CX, RCA all the Dmean and Dmax values were significantly superior in the d-IMRT plans, while there were no differences between the s-IMRT and the 3D-CRT plans (Table 1).
In the subgroup analysis, the patients were divided into two groups as node-positive (n = 11) and node-negative (n = 9) and reanalysed.
Subgroup analysis for the heart substructures
LA Dmax was not different between the three techniques in the node-positive group, whilst it was significantly superior in the d-IMRT plan in the node-negative group and no difference between s-IMRT and 3D-CRT techniques (the details are shown in Table 2). There were no differences between the three techniques for RA and LV Dmean, RV V45 values in the node-negative group, whereas in the node-positive group the d-IMRT technique was statistically superior to the s-IMRT and the 3D-CRT techniques (no difference in the s-IMRT and the 3D-CRT plans) (Table 2). In the remaining parameters, there were no statistical differences between the node-positive and negative groups.
Table 2. Statistically significant different parameters and mean ± standard deviation (SD) values between node-positive and negative groups

Abbreviations: L, left; R, right; LMCA, left main coronary artery; RCA, right coronary artery; Dmean, mean dose; Dmax, maximum dose; V45, the percentage of volume receiving 45 Gy; 3D-CRT, three-dimensional conformal radiotherapy; s-IMRT, static-intensity-modulated radiotherapy; d-IMRT, dynamic-intensity-modulated radiotherapy.
Bold values indicate statistical significance (p < 0.05).
Subgroup analysis for the coronary arteries
There were no differences between the three techniques for LMCA Dmax, RCA Dmean and RCA Dmax values in the node-negative groups. Nevertheless, the d-IMRT technique was significantly better in the node-positive group for all coronary arteries (Table 2).
Discussion
In this era of immunotherapy, the survival expectancy is prolonged, and the dose-limit information of the heart substructures and coronary vessels exposed to radiation is limited. In this study, the doses of the heart substructures and coronary arteries were investigated using different techniques during the RT of patients with lung cancer. Especially, those with central tumours were selected for the study. There are no other studies in the literature in which tumour areas are defined as clearly as ours. At the same time, the dosimetric effect of node-positive mediastinal irradiation on cardiac substructures with different RT techniques was also investigated. The d-IMRT plans were statistically the best optimal plan for PTV (Dmean, Dmax, V95, V107, CI and HI). The s-IMRT plans were significantly superior to 3D-CRT for PTV. RA Dmax and V45 were not different between the three techniques. The d-IMRT technique was significantly better in other heart substructures and coronary arteries. In the subgroup analysis, there was no difference between the techniques in the node-negative group [only the LA Dmax was the best in the d-IMRT technique, which barely reached the limit of significance (p = 0·045)]. The Dmean of RA, and LV, V45 of RV and coronary of LMCA Dmax, RCA Dmean, and RCA Dmax were significantly better in d-IMRT technique in the node-positive group, irradiated in the mediastinum. In the remaining parameters, there were no statistical differences between the node-positive and negative groups.
Nowadays, there is no consensus on the contouring of heart substructures or coronary arteries inRT. Currently, in practice, the whole heart is contoured as an organ at risk (OAR), and only this OAR is evaluated to predict cardiotoxicity in dose-volume histograms. However, there are many literatures proving the dose relationship between cardiotoxicity and cardiac substructures, especially concerning breast cancer. Due to the long life expectancy in lung cancer, heart substructures and coronary vessels should be protected with great precision. Recently, so many studies have been reported regarding this subject. Reference Jang, Cha and Kim12–Reference Chan, Ruan, Shaverdian, Raghavan, Cao and Lee17 In a recent study, the incidence of cardiac events related to the dose of cardiac substructures was evaluated in 258 patients with stage 3 NSCLC who underwent chemoradiotherapy. Especially, a LV volume receiving 60 Gy (LV V60) to be >0 was found to be significantly associated with the acute coronary syndrome. Reference Jang, Cha and Kim12 Wang et al. Reference Wang, Pearlstein and Patchett13 analysed 112 patients with stage 3 NSCLC who received dose-escalated RT (50–70 Gy) with a median follow-up of 8·8 years. The doses of LV were associated with ischemic cardiac events. The relationship between the doses of LA and RA and pericardial events has been demonstrated. Reference Wang, Pearlstein and Patchett13 In the study of Borkenhagen et al. Reference Borkenhagen, Bergom, Rapp, Klawikowski, Rein and Gore14 conducted with 76 patients with NCSLC who underwent thoracic RT, high ventricular volume receiving 45 Gy was found to be associated with post-RT cardiotoxicity. Reference Borkenhagen, Bergom, Rapp, Klawikowski, Rein and Gore14 In the study reported by Atkins et al. Reference Atkins, Chaunzwa and Lamba15 , cardiac substructures were delineated in 701 locally advanced NCSLC patients undergoing thoracic RT. They reported that LAD V15 Gy parameter equal to or greater than 10% increases the risk of major adverse cardiac events and mortality. Even if, the mean dose of the total coronary artery is 7 Gy or more in patients without cardiac disease, the 2-year mortality rates elevated significantly. Reference Atkins, Chaunzwa and Lamba15 In another study, they have examined the relationship of whole heart and substructures with symptomatic cardiac events, dosimetrically, in an inoperable 140 NSCLC patients who received 60–66 Gy RT. They found that the 4-year cumulative incidence of symptomatic cardiac events was 48·6% versus 18·5% for mean whole heart dose ≥ 20 Gy versus <20 Gy, respectively. Reference Yegya-Raman, Wang and Kim16 Chan et al. Reference Chan, Ruan, Shaverdian, Raghavan, Cao and Lee17 examined the effects of heart and substructure doses on survival in 153 patients with early-stage NSCLC who underwent stereotactic ablative RT. They reported that the most predictive parameter for overall survival was RV V10Gy. Indeed, RV V10Gy < 4% had significantly longer survival. Reference Chan, Ruan, Shaverdian, Raghavan, Cao and Lee17
In the study of Ferris et al. Reference Ferris, Martin and Switchenko18 , which is a dosimetric study like ours, they compared 26 locally advanced NSCLC patients for the preservation of cardiac substructures with regard to original volumetric-modulated arc therapy (VMAT)-optimised VMAT and intensity-modulated proton therapy (IMPT) techniques. As OARs, RA-LA ventricles, all coronary arteries and heart valves were delineated. As a result of the study, all heart substructures were found to be significantly better in the new cardiac-optimised VMAT plans compared to the original VMAT plans. On the other hand, the IMPT technique has been shown to provide just a few dosimetric improvements beyond the new cardiac-optimized VMAT technique, the clinical significance of which remains unclear. Reference Ferris, Martin and Switchenko18 The advanced RT techniques, such as VMAT and IMPT, are new and high-level techniques that are difficult to reach in rural areas in developing countries. In the oncology centres, located in rural areas with smaller populations, more traditional RT techniques are employed with more basic RT devices. The centre where our study was carried out was also as mentioned. Therefore, we designed a dosimetric study comparing s-IMRT (field-in-field) and a-IMRT techniques, which are more commonly used in such centres.
There were some limitations of our study. The simulation CT, where the plans were rendered, was not four-dimensional (plus respiratory controlled), that is, the target volume was determined without respiratory monitoring. The PTV margin for organ movement in the study was the same in all three techniques, because the dose was prescribed for the same PTV to ensure similarity between techniques. Since the MLC is mobile in the d-IMRT technique, it would be correct to actually irradiate with the Real-time Position Management (RPM) RT technique. As our study was dosimetric, the risk of cardiotoxicity related to the dose of the heart substructures could not be established.
Conclusion
In lung cancer RT, when a primary central tumur or mediastinal irradiation due to node positivity is carried out, heart substructures receive a substantial amount of dose. With different techniques, the doses to which these structures are exposed may vary. In our study, compared to 3D-CRT and s-IMRT techniques, the d-IMRT technique provided the best protection in all other heart substructures except for a few parameters (RA Dmax and V45 doses), while a homogeneous and conformal dose was supplied at the target volume.
Financial support
There are no financial support received
Conflicts of interest
No conflict of interest.
Ethical standards
The Departmental Ethics Committee of University of Tokat Gaziosmanpaşa, Faculty of Medicine Hospital approved this study.