Introduction
Bovine tuberculosis (bTB) is a chronic disease of livestock caused mainly by Mycobacterium bovis (M. bovis), with M. bovis infection also a risk to wildlife and humans. bTB is important economically, with impacts on both animal productivity and international trade [Reference Ayele1–Reference Tschopp6]. The importance of the disease has been increasing in developing countries where bTB is poorly controlled [Reference Cosivi7–Reference Hamzi, Mohd-Nor, Acosta and Sarmiento9]. In some industrial countries, bTB control in cattle has been achieved or at least the disease burden markedly reduced through the implementation of test and slaughter policies [10]. In some contexts, notably in the UK, Ireland and New Zealand, significant wildlife reservoirs contribute to disease spillover back to domesticated animals, hampering progress towards elimination [Reference Michel, Müller and van Helden11]. In Ethiopia, studies conducted so far have demonstrated that bTB is endemically established in its cattle population; however, there are no known wildlife reservoirs and the distribution of the disease is heterogeneous. Pastoral and crop–livestock production systems holding primarily Zebu cattle have lower animal prevalence (<10%) [Reference Romha12–Reference Gumi16] than herds in urban and peri-urban areas where genetically improved dairy cattle, mainly Holstein Friesian – Zebu crosses, are managed under intensive and semi-intensive management systems. These intensively managed herds have a higher prevalence and are mainly found in the central part of Ethiopia (11–47% animal prevalence) [Reference Ameni, Bonnet and Tibbo17–Reference Firdessa20]. An important driving factor for the spread of bTB is unregulated cattle movement/trading with poor biosecurity measures following the Ethiopian government initiatives and plans to expand and intensify the dairy industry in the country [Reference Mekonnen21]. Dairy farming is an emerging sector in the peripheral regions of the country, particularly in regional cities aiming to satisfy the demand for milk and milk products. The demand for dairy cattle in these areas is fulfilled by trade in areas where dairy farming is relatively well developed, both locally and in remote areas, but without prior knowledge as to the bTB status of the animals. Thus, the risk of spread of bTB and other production-related cattle diseases has become the constraining factor for the expansion of the industry.
The economic and public health risks of bTB in Ethiopia are assumed to be significant. For intensive dairy herds in Addis Ababa, infection with bTB has been estimated to cause a financial loss of 4–6% per year [Reference Tschopp22]. Condemnation of bTB-affected carcasses in slaughterhouses can also have an economic impact on the livelihoods of livestock keepers in Ethiopia [Reference Shitaye23]. In humans, the total incidence rate of tuberculosis in Ethiopia was 151 (107–204) per hundred thousand population in 2018 [24]. The prevalence of human tuberculosis due to M. bovis has been reported at 2.8% of all human tuberculosis cases globally, corresponding to about seven zoonotic tuberculosis cases per hundred thousand population per year [Reference Müller25]. In Ethiopia, although several publications have reported a relatively low prevalence of zoonotic tuberculosis, it is possible that the prevalence is higher among high-risk populations directly exposed to bTB-infected cattle or to unpasteurised dairy products [Reference Tibebu26–Reference Gumi28]. The need for controlling bTB to reduce the zoonotic risk and economic loss is, therefore, pressing.
Currently, there is no national bTB control programme for cattle in Ethiopia. Some intermittent attempts by individual farmers through voluntary removal or segregation of reactor cattle have been reported [Reference Ameni29]. Surveillance without statutory control on reactor animals has, in the view of the authors, become a challenge in the Ethiopian condition. Some farmers may be tempted to sell reactor cattle (G.A. Mekonnen, personal observation). This poses the risk of increasing bTB spread/transmission to other herds. Imposing veterinary regulatory procedures to reduce the risk of transmission is currently being considered by the Ethiopian government. In the present study, we conducted repeated tuberculin skin testing on herds in three regions of Ethiopia. We set out to assess the extent to which apparent prevalence is stable in the emerging dairy belts, to quantify the risk factors associated with transmission within herds using the subset of animals that became test-positive in the second test that were negative in the first test, and to assess the response of farmers to being informed of the reactor status of animals within their herds.
Materials and methods
Study sites
The study was conducted in three regional administrative cities, namely, Hawassa, Gondar and Mekelle in the southern, north-western and northern parts of Ethiopia, respectively. This choice was informed by the Ethiopian government strategic plan for dairy development, the three cities chosen to be representative geographically and of the modern dairy industry managed under intensive and semi-intensive systems. These cities are considered emerging dairy belts with relatively large dairy cattle populations (Table 1), and human populations of around 300 000 each [30].
Table 1. Dairy herds and cattle recruited for the 2016/17 and 2018 testing scheme

Study population
The study population was dairy cattle managed in the selected herds at the study sites. The dairy cattle (cattle reared for the purpose of milk production) in the selected herds were study units. The majority of animals were crosses of Holstein, Friesian and Zebu breeds (96.4%), with a few crosses of Jersey and Zebu (1.9%), or pure Zebu (1.7%). The management and setting of farms differed depending on the farmers’ experience and level of knowledge of dairy farming.
Study design and sampling strategy
The study design was cross-sectional with skin testing being repeated at a 1–2 years interval. The first round of testing was carried out across the years 2016–2017 and all tests in the second round were carried out within 2018. The sampling strategy was a one-stage cluster design, considering dairy herds as clusters and individual cattle as sampling units. Sample size calculations were described previously [Reference Mekonnen31]. The number of dairy herds computed and the corresponding number of cattle within the herds are shown in Table 1. During the first round of testing in 2016/17, herds with more than 20 animals were selected without any prerequisite, while herds with fewer than 20 animals were recruited using a random selection method among the complete list of dairy herds in the area. In 2018, every possible effort was made to retest the same herds selected in 2016/17. However, only a subset of herds could be retested as some refused to participate and a number of herds with high bTB burden were dissolved or changed to other types of businesses. In total, 26% (46/174) of the herds tested in the first survey were not included in the retest. To easily identify individual cattle, in the second round of testing, six digit ear tags were applied as an identifier for all cattle that had no owner-provided ear tag during the first survey. In both rounds of testing, all cattle except calves younger than 4 weeks, clinically sick cattle with disease not suggestive of bTB and cows in the last month of pregnancy were included. These inclusion criteria were set to avoid possible interference with the action of tuberculin [Reference de la Rua-Domenech8, Reference Goodchild and Clifton-Hadley32]. During the first round of survey, farmers were all informed of the reactor status of their cattle and relevant advice was forwarded on how to reduce the risk of bTB transmission such as removal of reactor cattle, avoiding contact with neighbour herds or new cattle of unknown bTB status.
Skin testing
The Single Intradermal Comparative Cervical Tuberculin (SICCT) test method was used to determine the bTB reactor status of cattle. The test was applied as described previously [Reference Mekonnen31]. Briefly, two sites in the middle third of the neck were shaved 12–15 cm apart parallel to the shoulder and the skin fold thickness measured. Animals were then inoculated with 0.1 ml (2500 IU/ml) avian PPD and 0.1 ml (3000 IU/ml) bovine PPD (Lelystad B.V., The Netherlands) in the prepared sites. The status of the tuberculin reaction was examined and the skin thickness was measured 72(±4) h after the first injection. A reaction was considered positive if the increase in skin thickness at the bovine site of injection was more than 4 mm greater than the reaction shown at the site of the avian injection, inconclusive if the increase was from 1 to 4 mm, or negative if the increase was <1 mm [33].
Questionnaire survey
Information on herd structure and risk factors was collected during the retesting in 2018 using a modified questionnaire employed previously [Reference Mekonnen31]. Risk factors considered in this study were identified from the literature [Reference Humblet, Boschiroli and Saegerman5, Reference Sibhat19]; a complete list of these factors was published [Reference Mekonnen31], a subset of the risk factors that were assumed to have association was included in this survey. The questionnaire contained open-ended and closed questions and was filled in by one researcher in all sites. Administration of the questionnaire was made using local language and in a way that the respondents felt easy. The objective and possible outcome of the study were explained and the respondents were told they could terminate the interview at any stage. As part of the survey, the bTB testing team treated sick animals with antibiotics, anthelminthic drugs and wound spray as incentives and advised owners to seek further advice from the local veterinary services.
Ethical considerations
Ethical approval to implement the research was granted by the Institutional Review Board (IRB) of Aklilu Lemma Institute of Pathobiology, Addis Ababa University (Reference number IRB/ALIPB/2018). This study was supported by the Ethiopian Ministry of Agriculture.
Data analysis
R statistical software (version 3.5.1) (R Core Team) and RStudio were used for all data analyses and modelling. SICCT test results and risk factor data collected from the subset of herds that were tested twice were examined. Apparent prevalence was calculated using the proportion of test-positives among all tested herds or animals, while the incidence rate between the two tests was defined as the number of cases (new test positive cattle or herds) per 100 cattle (or herd)-years. Time at risk, as measured herd-years required for herd-level incidence rate calculation, was the average follow-up period of the cattle in the herds. For the animal-level incidence rate, the animal-year was the summation of the time intervals between the two SICCT tests of the retested animals and the age of all calves born between surveys. In the incidence rate estimation, new infections were assumed to occur at the midpoint of the follow-up period.
The sub-group of animals with matched test results was used to explore individual animal risk factors for transmission in the interval between tests using generalised linear mixed models (GLMM) with a binomial response (logit link) and estimated using maximum likelihood (Adaptive Gauss-Hermite Quadrature) using the ‘lme4’ package [Reference Bates34]. Risk factors for inclusion in the final multivariable model were selected using a univariable screen, retaining variables with P < 0.20.
We estimated a herd-level random intercept to adjust for the heterogeneity in transmission risk between herds. Given the variability in apparent prevalence both within and between regions, we first considered a nested random effect (herd nested within the region). However, this model is singular and following Barr et al. [Reference Barr35], we simplified the model to include only a herd-level random effect. The random effect was tested by comparing the likelihood ratios of the models with and without the random effects and the difference was confirmed to be significant (P < 0.001) by the χ 2 test [Reference Gelman and Hill36]. The variance inflation factor was calculated to check the multicollinearity terms of the model using the package ‘performance’ [Reference Lüdecke, Makowski and Waggoner37]. Model fit was assessed by Hosmer and Lemeshow goodness of fit test using ‘resourceSelection’ package [Reference Lele, Keim and Solymos38]. Finally, the classification capability of the model was checked by the receiver operating characteristic curve (ROC) using ‘pROC’ package [Reference Robin39]. Package ‘aod’ [Reference Lesnoff and Lancelot40] and ‘questioner’ [Reference Barnier, Briatte and Larmarange41] were used to calculate the odds ratio (OR) and confidence intervals (CIs) in the GLMM and GLM, respectively. CIs for apparent prevalence and incidence rate were calculated using ‘EpiTools epidemiological calculators’ with Wilson method [Reference Sergeant42] and ‘OpenEpi’ with Fisher's exact test [Reference Dean, Sullivan and Soe43], respectively. In all cases, a 95% confidence level and a significance level of 5% were used to determine statistical significance.
Results
The apparent prevalence of bTB as measured by the SICCT test for our matched herds is summarised in Table 2, stratified by region and round of testing. A total of 128 herds were tested twice. The numbers of cattle tested in the two rounds were more or less similar, with 2294 cattle tested in 2016/17 and 2317 in 2018. This reflects the balance between the number of cattle removed between the two time points and the number of calves born (n = 624) and newly introduced (n = 194).
Table 2. Change in apparent prevalence between survey years and study sites (number of herds retested = 128, number of animals tested in 2016/17 = 2294, in 2018 = 2317)

AP, apparent prevalence; TP, true prevalence. At Sp of 100% TP estimate (a) 2.8 (1.6, 4.8); (b) 1.9 (1, 3.7). TP less than zero implies that the true prevalence cannot be distinguished from zero based on the assumed test characteristics. *Within-herd apparent prevalence was calculated only for herds with at least one tuberculin reactor detected in the respective survey years.
GLM regression analysis was done to assess if herds not included in the retest (26%, 45/174) were associated with their SICCT test reactor status disclosed in the first test. The model output showed that herds reduced from the retest due to refusal to participation or dissolved herds or change of farming businesses were not statistically significantly associated with the bTB positivity (OR 0.63, 95% CI 0.25–1.7, P > 0.05). At the animal-level, 35% (802/2317) of the animals tested in 2018 could not be paired with animals tested in 2016/17 due to lost or changed ear tags. To assess the unpaired rate (assumed to mirror the removal rate) between reactors and non-reactors classified by the first test, a regression analysis using GLMM with herd ID as a random effect was done by adjusting with age to account for the background removal rate. The model output showed that the removal rate in the reactor group of animals was three times higher compared to the removal rate in non-reactors (OR 3.0, 95% CI 1.4–7). However, both the animal and herd-level apparent prevalence were consistent between the two surveys, with no significant difference between the two time points and a consistent (and statistically significant) difference between the study sites.
Incidence rate
Eight hundred and eighty-six animals belonging to 107 herds were matched between the 2016/17 and 2018 SICCT herd tests using ear tags. The majority of matched animals were SICCT-negative in both tests (n = 810), 15 cattle were tested positive in both surveys, 57 became positive in the second test, and four were positive in the first test but tested negative in the second SICCT test. These four animals were all relatively strong reactors in the first test (bovine PPD measurements of 9–22 mm, Table 3). Two would have been classified as reactors under the single intradermal tuberculin test with a skin thickness increase >4 mm, but all four demonstrated a considerable reduction in their response to bovine PPD of ~10 mm between the two tests. To estimate the overall incidence rate at animal-level, a subset of paired cattle that were SICCT test-negative in the first survey (n = 867) and calves born between the two surveys (n = 624) were used to estimate the incidence rate of bTB positivity and assess the risk of transmission within herds. Accordingly, the animal-level incidence rate was estimated at 3.6/100 animal-years (Table 4). The incidence rate in Mekelle and Hawassa was significantly higher compared to that in Gondar (P < 0.05). The higher incidence of bTB positivity in Mekelle suggested a higher risk of within-herd transmission as would be expected given the higher apparent prevalence of reactors in the first test. In Hawassa, although the overall apparent prevalence in the 2016/17 survey was lower (see Table 2), an overall higher incidence rate was observed due to the contribution of a single large farm (n = 113). Although this farm attempted to segregate or remove reactor animals identified in the first round, there was a twofold increase in the within-herd apparent prevalence (21%, 24/113, first survey to 47%, 68/145, retest).
Table 3. Skin test reading of animals that were positive by first survey becoming negative in the second survey

Δ – Greek letter delta; ΔA and ΔB – difference in measurements at time 0 and 72 h post-PPD injection of the avian PPD and bovine PPD, respectively; while ΔBA stands for the difference of the measurement increases at the bovine and avian sites after 72 h post-injections.
Table 4. Estimated incidence rate of bTB reactors based on the number of new reactors in 2018 among herds and animals

Herd-years – calculated using average follow-up year(s) of the animals in the herds (Gondar – 1 year, Hawassa – 1.37 years and Mekelle – 1.57 years); animal-years – summation of the time interval between the two SICCT tests for the retested cattle and age of calves born between surveys were considered; new infection was assumed to occur at the mid of the follow-up period and this was considered in the calculations; n – stands for number of herds or animals under investigation. Herds and animals considered for incidence rate estimation were all SICCT-negative in the 2016/17 test.
At the herd-level, 24% (31/128, 95% CI 17.5–32%) of the retested herds were positive in the first survey, while the remaining were classified negative by the SICCT test. Out of the negative herds in the 2016/17 test, the herd-level incidence rate of bTB positivity was 6.6 (95% CI 3.0–12.6) per 100 herd-years (Table 4).
Risk factors associated with bTB transmission
The detection of animals switching from negative to positive SICCT status between tests provides an opportunity to explore risk factors for transmission over this period. GLMM was used to adjust for clustering effects within herds. The model fit and classification capability of the GLMM were evaluated as described above (methods). The Hosmer and Lemeshow goodness of fit revealed no evidence of a systematic lack of fit of the model (χ 2 = 7.76, df = 8, P = 0.46). The classification ability of the model as evaluated by ROC analysis is excellent (area under the curve 0.96). Exposure time, age group, herd size, herd risk and sex were selected by the univariable screen. Herd risk was categorised based on the within-herd apparent prevalence according to the SICCT test result of the 2016/17 survey. Sex was dropped from the final model due to the small number of males, which were normally removed from the herd by the owners at an early age to reduce maintaining cost. The univariable screen also identified calves born in between the surveys as having a lower risk of being a reactor (OR 0.4, 95% CI 0.2–0.7). However, this variable was omitted from the final multivariable model to avoid its confounding effect with age.
The estimated risk factors from the final multivariable model are presented in Table 5. Age and herd risk were the only explanatory factors to have a significant association with the risk of becoming a reactor, while exposure time and herd size had a large effect size but were not statistically significant. The pattern of incidence of SICCT test positivity across age groups appeared to increase with the peak risk between the age of 5 and 7 years.
Table 5. Risk factors from multivariable GLMM model for the incidence of bTB reactor status (number of animal = 1491; number of herds = 128; 2 missing on animal data)
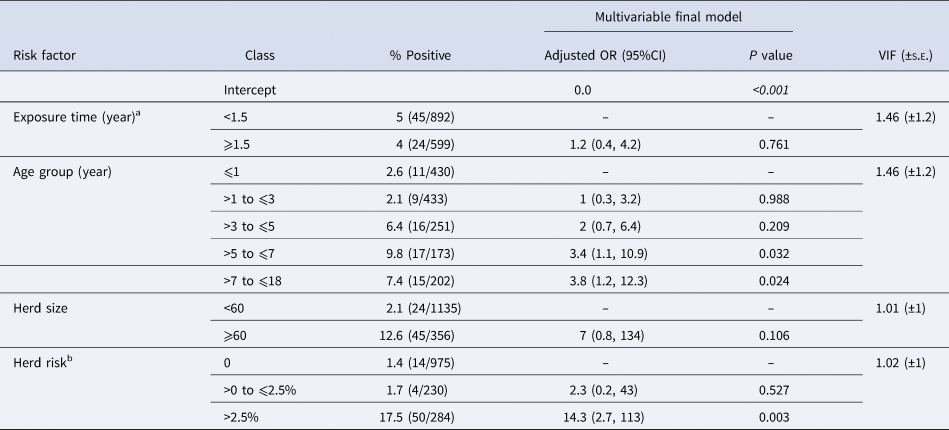
OR, odds ratio; VIF, variance inflation factor; s.e., standard error.
a Exposure time refers to the time period the animals/herds were followed.
b Herd risk was categorised based on the within-herd apparent prevalence according to the SICCT test status from the 2016/17 survey (category 1: herds with 0 apparent prevalence, category 2: herds with apparent prevalence between 0% and 2.5%, category 3: herds with apparent prevalence of >2.5%).
Farmers’ response to disclosure of reactor status of their cattle
Following the first survey in 2016/17, farmers were advised to remove reactor cattle to reduce transmission and clearing herds of bTB through time. SICCT test results of the 2018 survey comparing herds that removed reactors and herds that did not remove reactors are summarised in Table 6. Among the retested herds in the 2018 survey (n = 128), 31 were tested positive in 2016/17: of these, reactor cattle were removed from 18 herds following disclosure of reactor status; while no reactors were removed from 13 herds.
Table 6. Comparison of herd and animal-level bTB apparent prevalence following removal of SICCT test reactor cattle from herds that were positive in the 2016/17 test (number of herds = 31, number of animals = 751)

In herds where reactors were removed, the average within-herd apparent prevalence was decreased by 75% (within-herd apparent prevalence in 2016/17, 9.3% (95% CI 6.7–11.9); in 2018, 2.3% (95% CI 0–4.7)); however, in herds where reactors were partially or totally not removed, the average within-herd apparent prevalence increased marginally by 8.3% (within-herd apparent prevalence in 2016/17, 25.3% (95% CI 12.2–38.4); in 2018, 27.4% (95% CI 11.3–43.5)).
Considering only the sub-population of cattle that were SICCT test-negative in the 2016/17 survey (and calves born in between survey years), the incidence rate in herds that did not remove reactors was higher (16.6 per 100 animal-years, animal-years 259) compared to herds that removed reactors (3 per 100 animal-years, animal-years 365) following the disclosure of reactor status of the cattle. This corresponds to an 82% difference in the incidence rate on these herds compared to those that did not remove reactors.
Discussion
In Ethiopia, a lower apparent prevalence of bTB has been reported in dairies in peripheral regions as compared to the highly infected dairy belts in central Ethiopia [Reference Firdessa20], and this raises the question as to whether these populations are endemically infected at a lower level than the dairy herds in central Ethiopia or these herds are merely at an earlier stage of infection. To address this question, we carried out a second round of testing on herds previously screened for bTB to assess how apparent prevalence within the herds changed and to identify risk factors associated with the detection of new reactor animals. As the status of animals was disclosed to farmers during the first round of testing, we also had an opportunity to assess the effect of farmers’ response to this knowledge and the impact this had on the risk of transmission within their herds.
Herd- and animal-level apparent prevalence and incidence rate
In this study, there was no statistically significant difference in the overall apparent prevalence of bTB between surveys. Despite the active steps taken to remove reactors from some herds, which could have caused some level of selection bias, the loss of reactor animals was effectively balanced by the detection of new reactors on the second test. While this is suggestive of an endemically infected population, the rate of increase in apparent prevalence may simply be too slow to detect given the relatively short period between tests and the size of our sample.
Comparing between study sites, there were some intriguing regional differences and outlier herds that warrant further discussion. The differences in apparent prevalence between study sites were consistent between the two surveys, with apparent prevalence in Mekelle still significantly higher than the other two regions. In line with this difference in apparent prevalence, the number of new reactors in the second round of testing was higher in Mekelle and Hawassa than in Gondar. This could simply be related to the presence of a higher proportion of infected herds and animals with little or no control effort being done following the disclosure of the 2016/17 SICCT test results.
In Hawassa, a higher animal-level incidence rate of SICCT test positivity suggests a higher rate of within-herd transmission from reactors that were not removed from the herds. The difference was driven by a single large herd (n = 113), which attempted to introduce control through the segregation of reactor animals. However, segregation was poorly achieved, which might have contributed to the twofold increase in the number of reactors over the course of a single year. Historical data for this specific herd showed that the herd was first infected following a new cattle introduction in the period between 2014 and 2015 (NAHDIC, unpublished data). Unlike the experience in this herd, proper segregation practice has been suggested to reduce the incidence rate of reactor status. In this regard, Ameni et al. [Reference Ameni29] reported a reduction of incidence from 14 cases to one case/100 cattle/year after three consecutive test-and-segregation exercises. However, the practicality of this strategy in Ethiopia may be limited as it highly depends on the availability of sufficient space and commitment to completely isolate bTB reactors from SICCT test-negative animals.
Risk factors for transmission within herds
A key motivation for the repeated testing of our study herds was to attempt to quantify the rate of transmission of bTB and associated risk factors to inform mathematical transmission modelling of control. To this end, we carried out a risk factor analysis using a specific dataset of cattle negative in the 2016/17 survey and calves born in between the surveys to identify risk factors associated with the detection of new reactor animals. We found that age group and herd risk were significantly associated with animals becoming reactors to the SICCT test.
The present findings and others [Reference Reynolds44–Reference Francis47] suggest age affects the probability that an animal tests positive and transmission risk increases with age due to a higher probability of contact and/or prolonged exposure to other infected animals or environmental contamination. The magnitude of the within-herd bTB apparent prevalence in the first test increased the risk of animals becoming reactors in the second survey. Cattle kept in herds with apparent prevalence >2.5% were more likely to be reactors in the second survey than those kept in negative herds or herds with apparent prevalence below 2.5% (Table 5). This is probably due to the presence of a larger number of infected animals in these herds serving as sources of infection for non-infected animals, the rate of which can indeed be facilitated in poorly managed herds [Reference More and Good48, Reference Clegg49].
However, these results must be carefully interpreted with respect to key limitations of our study and the quality of the data we were able to collect. The SICCT test has a relatively high specificity [Reference Goodchild50], but low sensitivity [Reference de la Rua-Domenech8, Reference Karolemeas51, Reference Monaghan52], so the true prevalence of bTB is likely to be considerably higher than the apparent prevalence. Furthermore, new reactor animals identified in the second round may include animals missed by the first round of testing (specifically cattle in an early stage of infection, intercurrent, especially parasitic infections, and/or temporary anergic state like recent parturition during the first survey), thus increasing the apparent rate of transmission between animals. The use of ancillary test methods, such as the interferon γ test in combination with the SICCT test could have increased sensitivity (albeit at the expense of specificity) [53]. This was unfortunately not possible in this study due to the remoteness of the study populations from suitable laboratory facilities, a key challenge in the context of less developed countries.
Another important limitation of our dataset is the potential that selection bias has been introduced in our sample of matched animals/herds between tests. We were only able to pair about 52% of cattle present in the first round during the second round of testing, despite efforts to ensure the identifiability of animals. We applied ear tags to all cattle that had no identification (ID) in the first round of testing. However, some of these IDs were replaced by farmers between visits by a new national ID introduced by the Ethiopian government to establish a national-level dairy database. IDs used by farmers (cattle names) were found not to be reliable and prone to change when animal attendants leave the farm as no written records were kept. Such problems are expected to be a challenge in developing countries, particularly in situations where there is no national identification and traceability system. With regards to the SICCT test, the interpretation of the results used in this study was according to the recommended OIE standard method [33]; however, the estimate for animal-level apparent prevalence is likely imperfect due to the considerable uncertainty of the test sensitivity and specificity for bTB diagnosis. Sensitivity and specificity for the SICCT test in Ethiopian herds have been estimated as 59% (95% CI 49–69%) and 97% (95% CI 89–100%), respectively [Reference Ameni54]. The estimate for animal-level true prevalence in the first and second rounds of testing using the point estimates from this study was 2.7% (95% CI 1.3–4.3%) and 3.6% (95% CI 2.1–5.3%), respectively (Table 2), slightly lower than the apparent prevalence suggesting a relatively lower specificity of the test. The negative ‘true prevalence’ estimates in Gondar for animal-level prevalence are an artefact of the sample size and small number of affected herds – with high confidence that the true within-herd prevalence in this area is great than zero. Estimates of true prevalence should also be cautiously interpreted given the uncertainty in test characteristics. In particular, studies for the UK based on orders of magnitude more animals have suggested an exceptionally high specificity for the comparative test [Reference Nuñez-Garcia55] of up to 100%.
The tuberculin used in the two rounds of testing was not of the same batch although produced by the same company. This might have caused some discrepancy in the SICCT test results [Reference Downs56]; thus, in future studies, we would recommend the use of the same batch of tuberculin in multiple measurements, combining the SICCT test with other more sensitive test methods, such as the defined antigen skin test (DST) [Reference Srinivasan57], to identify infected cattle with false-negative test results. We also recommend applying ear tags, such as ear notches or photographs of animals to mitigate the identification issues of cattle and the potential confounding that they may introduce.
Farmers’ response to disclosure of reactor status and potential impacts on control
Despite the challenges in matching animals between tests, our supplemental questionnaire data demonstrate that some farmers choose to remove reactor animals from their herds, which was associated with a reduced risk of new reactors being detected to the herd over a comparatively short time-scale of 1–2 years.
This study shows that the voluntary removal of SICCT test reactor cattle by farmers would be a likely consequence of the survey implemented. Even though our analysis suggests this, it could lead to a reduction in the rate of transmission within these herds; however, if these animals are not slaughtered but sold to other herds, it could increase transmission between herds, given that there is no current legislative barrier to trading reactor animals. The Ethiopian government is currently considering establishing a systematic bTB surveillance programme in government-owned herds. Our results illustrate the need to carefully consider the regulation of sales of reactor animals as part of any initiative and the need for careful monitoring of the impacts as any nascent surveillance programme is rolled out.
In conclusion, the present study showed stable bTB apparent prevalence between surveys despite systematic and consistent differences in prevalence between regions. We found that the risk of transmission within herds was most strongly related to the age of animals and the within-herd apparent prevalence. Voluntary action towards the removal of reactor cattle should be encouraged, but there is currently a statutory gap with respect to preventing the potential increased risks of onward transmission between herds, which requires attention in the future.
Acknowledgements
The authors are indebted to the management of the National Animal Health Diagnostic and Investigation Centre (NAHDIC) and its technical staff who supported the field testing and data collection; Regional Agricultural Bureaus or Livestock Agencies, Dairy co-operations and Dairy farmers who collaborated or allowed this work to be done. We would also like to extend our appreciations to the editors and reviewers for their contribution to shape the manuscript.
Author contributions
GAM and AJKC conceived and designed the research, contributed equally to data analysis and write up. SB, JLNW, AM and GA conceived and designed the research; GAM, HA and AO performed the research; GAM, AJKC and BTA analysed the data; GAM and AJKC wrote the manuscript; SB, BTA, AM, GA and JLNW critically reviewed the manuscript.
Financial support
This work was funded by the Biotechnology and Biological Sciences Research Council, the Department for International Development, the Economic & Social Research Council, the Medical Research Council, the Natural Environment Research Council and the Defence Science & Technology Laboratory, under the Zoonoses and Emerging Livestock Systems (ZELS) programme, ref: BB/L018977/1. Stefan Berg was also funded by Defra, UK, ref: TBSE3294.
Conflict of interest
None.
Data availability statement
All data generated and/or analysed during the current study are not publicly available but are available from the corresponding author on reasonable request.