Impact Statements
The pollution of water and waterways from land-based human activities has extensive impacts on both human and ecosystem health, contributing to significant global health burdens and loss of critical ecosystem services. Management of pollution is therefore a major focus of multiple sustainable development goals (SDGs) to achieve targets for: zero hunger (SDG 2); good health and well-being (SDG 3); clean water and sanitation (SDG 6); climate action (SDG 13); life below water (SDG 14); and life on land (SDG 15). Despite extensive and complex impacts of poor water quality, pollution control has been highly sectorised and under-resourced, with poor coordination of implementation, often across insufficient scales to realise benefits. This review provides a novel summary of the overlapping impacts of water pollution to downstream public and coastal ecosystem health to support planning and decision-making that benefits a wide range of stakeholders from government, civil society and the private sector. We provide evidence-based suggestions to optimise investments in holistic, integrated watershed management (IWM) to improve water quality and achieve overall systems health, which also provides co-benefits for biodiversity and climate. We also identify the key enabling factors required to coordinate and monitor IWM implementation to achieve desired outcomes. Specifically, the summary of pollution impacts and suggested management strategies provided in this review aim to provide awareness and tools to alleviate impacts to nutrition, water-related disease burdens and food poisoning that arise from poor water quality, which cause devastating economic and health costs disproportionately borne by the poorest countries.
Introduction
Tropical coastal ecosystems support some of the most diverse and productive environments on Earth and provide millions of people with vital ecosystem goods and services, such as food, livelihoods and coastal protection (Moberg and Folke, Reference Moberg and Folke1999; Cesar et al., Reference Cesar, Burke and Pet-Soede2003). However, with over 1.3 billion people in the tropics living within 100 km of coastlines (Sale et al., Reference Sale, Agardy, Ainsworth, Feist, Bell, Christie, Hoegh-Guldberg, Mumby, Feary, Saunders, Daw, Foale, Levin, Lindeman, Lorenzen, Pomeroy, Allison, Bradbury, Corrin, Edwards, Obura, Sadovy de Mitcheson, Samoilys and Sheppard2014), coastal ecosystems are becoming increasingly threatened by a suite of local, regional and global human activities, many of which affect water quality (Bellwood et al., Reference Bellwood, Hughes, Folke and Nyström2004; Lotze et al., Reference Lotze, Lenihan, Bourque, Bradbury, Cooke, Kay, Kidwell, Kirby, Peterson and Jackson2006; Orth et al., Reference Orth, Carruthers, Dennison, Duarte, Fourqurean, Heck, Hughes, Kendrick, Kenworthy, Olyarnik, Short, Waycott and Williams2006). Declining water quality is a primary driver of coastal ecosystem degradation (Crain et al., Reference Crain, Halpern, Beck and Kappel2009). Declines in water quality are driven mainly by pollutants from upstream human activities within watersheds flowing into coastal environments and are expected to worsen with increased coastal development and future climate change (Rabalais et al., Reference Rabalais, Turner, Díaz and Justić2009; He and Silliman, Reference He and Silliman2019).
Watershed management has received increasing focus as a tool for preserving the health of downstream coastal ecosystems, with research demonstrating critical land–sea linkages for coastal ecosystem health (Carlson et al., Reference Carlson, Foo and Asner2019; Sahavacharin et al., Reference Sahavacharin, Sompongchaiyakul and Thaitakoo2022). Despite the extensive literature and examples of decline, there are few examples of watershed management producing improvements to tropical coastal ecosystem conditions (Wear, Reference Wear2016). Challenges in achieving measurable success are largely due to the large spatial scale over which interventions often need to be applied within watersheds to adequately address multiple sources of pollution, capacity shortfalls for necessary monitoring, and the temporal lags to detect any changes in water quality and/or ecosystem health within coastal environments (Meals et al., Reference Meals, Dressing and Davenport2010).
Watershed condition also regulates a suite of processes that affect human health and wellbeing, including water filtration, flood management, and the provision of important cultural and recreational services (Jenkins et al., Reference Jenkins, Capon, Negin, Marais, Sorrell, Parkes and Horwitz2018a). Polluted water flowing within watersheds onto coastal environments is a major contributor to global human disease burdens, with poor water quality conservatively estimated to result annually in 1.4 million deaths, 3 million disability-adjusted life years and 12 billion USD in economic losses, a cost disproportionately borne by the poorest countries (Shuval, Reference Shuval2003; Fuller et al., Reference Fuller, Landrigan, Balakrishnan, Bathan, Bose-O’Reilly, Brauer, Caravanos, Chiles, Cohen, Corra, Cropper, Ferraro, Hanna, Hanrahan, Hu, Hunter, Janata, Kupka, Lanphear, Lichtveld, Martin, Mustapha, Sanchez-Triana, Sandilya, Schaefli, Shaw, Seddon, Suk, Téllez-Rojo and Yan2022). Yet the influence of watershed management on human health is rarely considered and is largely absent from public health literature (Bunch et al., Reference Bunch, Parkes, Zubrycki, Venema, Hallstrom, Neudorffer, Berbés-Blázquez and Morrison2014).
Identifying the overlapping upstream drivers of poor water quality that also create significant risks to public health presents an opportunity to motivate action and leverage long-term and large-scale investments while simultaneously improving coastal ecosystem water quality. By facilitating both human and ecosystem health, watersheds can serve as a focal area for place-based management interventions that serve to promote overall systems health (Cadham et al., Reference Cadham, Thomas, Khawlie and Kawass2005; Parkes and Horwitz, Reference Parkes and Horwitz2009; Jenkins et al., Reference Jenkins, Horwitz and Arabena2018b; Jordan and Benson, Reference Jordan and Benson2020). Here, we consider systems health as the emergent result of functioning interdependencies, interactions and feedbacks between ecological and socio-cultural settings, behaviour, and physiology, nested across micro-level (e.g., communities of microbes), meso-level (e.g., watersheds) and macro-level (e.g., global climate patterns) domains.
This review aims to: (1) synthesise and summarise the latest science regarding water quality impacts on coastal ecosystems (focused primarily on coral reefs); (2) identify pathways to improve systems health through policy implementation and direct management actions; and (3) provide evidence-based suggestions for strategic investments in watershed interventions across sectors that can help achieve multiple sustainable development goals (SDGs) and other global commitments and targets relating to biodiversity, marine pollution and public health.
Water quality impacts on coastal ecosystems
The quantity and quality of land-based runoff flowing into adjacent coastal ecosystems is determined by the characteristics of the watershed, such as geology, rainfall, soil type, land cover/vegetation (type and quantity) and slope (Douglas, Reference Douglas1967). There is a large body of evidence that demonstrates how human activities within watersheds alter runoff by removing native vegetation, changing the hydrology, altering microbial communities and adding/increasing pollutants within runoff (e.g., Peters and Meybeck, Reference Peters and Meybeck2000; Liao et al., Reference Liao, Yen, Guan, Ke and Liu2020).
Several broad pollutant categories are used to describe the pollutants reaching coastal waters from land-based activities. Here, we focus on the following common categories applicable to both human and coastal ecosystem health: sediments, nutrients, persistent organic pollutants (POPs), plastics and microdebris, pathogens, heavy metals, and pharmaceuticals and personal care products (Todd et al., Reference Todd, Ong and Chou2010; World Health Organization (WHO), 2016; Kroon et al., Reference Kroon, Berry, Brinkman, Kookana, Leusch, Melvin, Neale, Negri, Puotinen, Tsang and van de Merwe2020). Terrestrially derived sediments, heavy metals and nutrients are naturally transported from soils into coastal environments by ground and surface water, but due to large-scale human activities such as land-clearing (Table 1), the sources and transport into coastal waters has increased drastically, threatening over 30% of coral reefs globally (Andrello et al., Reference Andrello, Darling, Wenger, Suárez‐Castro, Gelfand and Ahmadia2021). POPs are synthetic organic chemicals that can persist in soils and water and bioaccumulate in organisms. POPs are widely produced across industries (Table 1) both intentionally, such as some insecticides, and unintentionally as by-products, such as dioxins (Weber et al., Reference Weber, de Beer, Lott, Polerecky, Kohls, Abed, Ferdelman and Fabricius2011). Other synthetic pollutants include the nonorganic plastics and microdebris, which can flow into coastal waters from numerous human sources (Table 1) such as trash, litter and weathering of materials like tires (Smith et al., Reference Smith, Love, Rochman and Neff2018; Macleod et al., Reference MacLeod, Arp, Tekman and Jahnke2021). Pathogens are disease-causing microbes and can naturally exist in coastal water and organisms but can also be introduced from land-based sources such as sewage (Table 1). Pharmaceuticals include chemicals used for personal, agricultural or animal health, such as antibiotics, while personal care products include chemicals generally used for cosmetic reasons, such as shampoos and moisturisers (Boxall et al., Reference Boxall, Rudd, Brooks, Caldwell, Choi, Hickmann, Innes, Ostapyk, Staveley, Verslycke and Ankley2012).
Table 1. Key references documenting global/regional linkages between human activities within watersheds and elevated levels of pollutants in runoff to coastal ecosystems

The primary land-based activities creating these pollutants and driving global declines in coastal water quality are land clearing, poor food production practices, urban development, mining and poor wastewater management (domestic and industrial) (Lu et al., Reference Lu, Yuan, Lu, Su, Zhang, Wang, Cao, Li, Su, Ittekkot, Garbutt, Bush, Fletcher, Wagey, Kachur and Sweijd2018). These human activities erode or release pollutants such as sediment, metals, pathogens and nutrients into surface and groundwater, which are then transported downstream to coastal environments (Crain et al., Reference Crain, Halpern, Beck and Kappel2009; Amato et al., Reference Amato, Bishop, Glenn, Dulai and Smith2016). The flow of impacts from human activities within watersheds to coastal ecosystems is summarised below (Figure 1).

Figure 1. Diagram depicting flow of impacts from key land-based activities on water quality properties that reach coral reef ecosystems.
As outlined in Table 1, pollutants can have multiple sources that can make it difficult to pinpoint which activity in a watershed is having the greatest impact on coastal ecosystems. For example, nutrients and sediments can originate from both wastewater pollution and agricultural runoff (Figure 1). Similarly, pharmaceuticals and personal care products can originate from cosmetics and medications used domestically as well as from medications used in agriculture (Table 1). In addition to the complexity of sources and types of pollutants, synergistic impacts and interactions occur when multiple pollutants are present at elevated levels, which can exacerbate the degradation of coastal ecosystems and harm associated organisms (Lu et al., Reference Lu, Yuan, Lu, Su, Zhang, Wang, Cao, Li, Su, Ittekkot, Garbutt, Bush, Fletcher, Wagey, Kachur and Sweijd2018; Huang et al., Reference Huang, Chen, Song, Deng, Shen, Chen, Zeng and Liang2021). Synergistic impacts and interactions also occur when pollutants are present with other stressors, such as climate change, disease, invasive species and overfishing. We focus on synergistic interactions on coral reefs, given the large body of research.
Herbivory is an important ecological process within coral reef ecosystems and can have complex and synergistic interactions with poor water quality (Table 2; Mumby et al., Reference Mumby, Hastings and Edwards2007). For example, in reefs with combined exposure to poor water quality and few herbivores, macroalgae and sediment-laden turfs can replace live coral as the dominant benthos (McField et al., Reference McField, Kramer, Giró Petersen, Soto, Drysdale, Craig and Rueda-Flores2020, Reference McField, Soto, Craig, Giró, Drysdale, Rueda-Flores, Castillo, Kramer and Roth2022). Sea level rise and climate-driven ocean warming are predicted to increase the sensitivity of coral reef ecosystems to poor water quality. Land-based pollution can lower the threshold for thermal stress and increase coral sensitivity to infection, resulting in increased bleaching (Fisher et al., Reference Fisher, Bessell-Browne and Jones2019), coral mortality (Claar et al., Reference Claar, Starko, Tietjen, Epstein, Cunning, Cobb, Baker, Gates and Baum2020) and outbreaks of disease on coral reefs (Vega-Thurber et al., Reference Vega-Thurber, Mydlarz, Brandt, Harvell, Weil, Raymundo, Willis, Langevin, Tracy and Littman2020). Corals that bleach from thermal stress also have reduced capacity to cope with sediment pollution (Bessell-Browne et al., Reference Bessell-Browne, Negri, Fisher, Clode and Jones2017). Nutrient pollution can result in brittle corals that are less resilient to the impacts of climate change, such as sea level rise and the increased severity and frequency of cyclones (Table 2; Rice et al., Reference Rice, Maher, Correa, Moeller, Lemoine, Shantz, Burkepile and Silbiger2020). Improving water quality through management of human activities within watersheds can therefore improve the resilience of corals to global impacts such as climate change.
Table 2. Impacts of poor water quality on humans, coral reefs, and coral reef organisms categorised by pollutant type, with key references indicated for further information
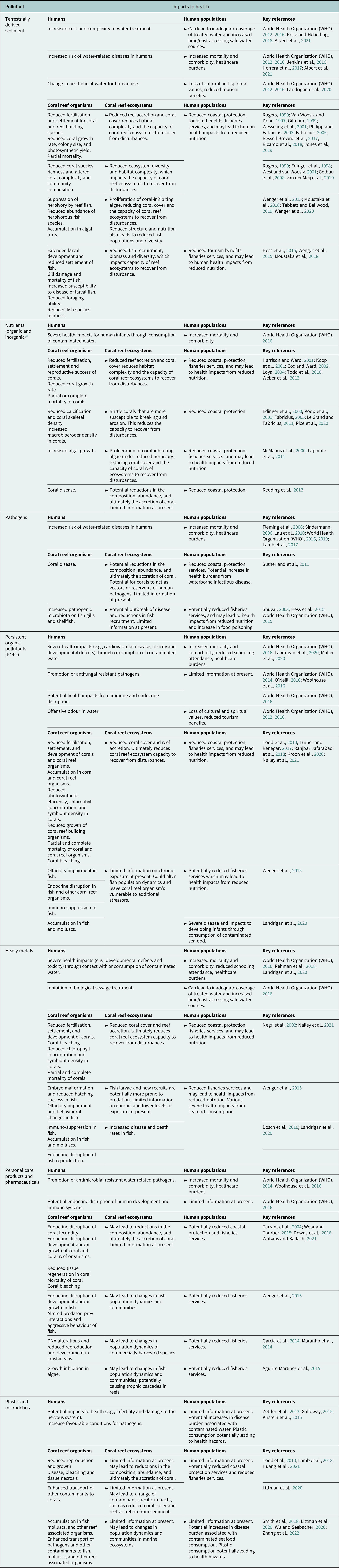
* Contaminant dynamics are complex, with different impacts and response curves observed even between contaminants in the same group (e.g., different heavy metals generate different impacts, different types of nutrients generate different impacts). Different levels of exposure also generate different responses, with some nutrient species generating positive responses under certain exposure levels. Impacts reported here are a general summary of known impacts from the introduction of each contaminant group at harmful levels observed in the environment.
Water quality impacts on human health
Many of the same drivers of declines in water quality and aquatic biodiversity, such as watershed deforestation, forest fragmentation on riverbanks and poor coverage of sanitation services, are also associated with human health impacts (Table 2). Impacts to humans from poor water quality include enhanced transmission of disease through polluted water and waterways, nutrition deficits from fisheries decline and chronic illness, and food poisoning from the contamination of important aquatic foods (Shuval, Reference Shuval2003; World Health Organization (WHO), 2015; Chase and Ngure, Reference Chase and Ngure2016). Over a million people die each year from water-related diseases, and at least 50% of these deaths are children and attributable to microbial intestinal infections (Kovacs et al., Reference Kovacs, Mullholland, Bosch, Campbell, Forouzanfar, Khalil, Lim, Liu, Maley, Mathers, Matheson, Mokdad, O’Brien, Parashar, Schaafsma, Steele, Hawes and Grove2015). Water related diseases such as diarrhoea are major contributors to global disease burdens, causing 8% of all deaths in children under the age of 5 years largely due to inadequate drinking-water quality (United Nations Inter-Agency Group for Child Mortality Estimation (UN IGME), 2019; World Health Organization (WHO) and United Nations Children’s Fund (UNICEF), 2021). Persistent endemicity and explosive outbreaks of water-related disease are often fuelled by interacting environmental factors related to climate change, land use and changing social conditions (Cann et al., Reference Cann, Thomas, Salmon, Wyn-Jones and Kay2013; Prüss-Ustün et al., Reference Prüss-Ustün, Wolf, Bartram, Clasen, Cumming, Freeman, Gordon, Hunter, Medlicott and Johnston2019). Water-related illness and travel associated with accessing safe water sources also contributes to reduced socioeconomic outcomes, such as reduced school attendance and gender equity (Fisher, Reference Fisher2008; Sorenson et al., Reference Sorenson, Morssink and Campos2011).
Communities reliant on surface and groundwater sources for drinking, bathing and household cleaning water are most at risk to water-related diseases and exposure to pollutants of emerging concern, particularly in tropical environments (Ragosta et al., Reference Ragosta, Evensen, Atwill, Walker, Ticktin, Asquith and Tate2011; World Health Organization (WHO), 2016; Herrera et al., Reference Herrera, Ellis, Fisher, Golden, Johnson, Mulligan, Pfaff, Treuer and Ricketts2017). Climate change is predicted to further increase global disease burdens by altering water-related disease dynamics (Semenza, Reference Semenza2020). Changes in rainfall and temperature will threaten water security, enhance pathogen survival and virulence, and increase exposure to contaminated water through multiple pathways, including flooding (Hofstra, Reference Hofstra2011; Levy et al., Reference Levy, Smith and Carlton2018). Rates of diarrhoea are predicted to increase under warmer and/or wetter conditions, with 1°C of warming predicted to increase diarrhoeal disease by 5% in developing countries (Singh et al., Reference Singh, Hales, De Wet, Raj, Hearnden and Weinstein2001).
Although water-related diseases are more often associated with exposure on land and freshwater, polluted seawater also presents a significant risk to human health. An estimated 180 million cases of upper respiratory disease and gastroenteritis occur each year due to humans bathing in polluted ocean waters or ingesting contaminated seafood, while around 4 million cases (and 40 thousand deaths) of infectious hepatitis A and E (HAV/HEV) occur annually from contaminated seafood from polluted coastal waters (Shuval, Reference Shuval2003; World Health Organization (WHO), 2015). Additionally, seafood contaminated with methylmercury and polychlorinated biphenyls can cause cardiovascular diseases in humans as well as severe impacts to infants in utero (Landrigan et al., Reference Landrigan, Stegeman, Fleming, Allemand, Anderson, Backer, Brucker-Davis, Chevalier, Corra, Czerucka, Bottein, Demeneix, Depledge, Deheyn, Dorman, Fénichel, Fisher, Gaill, Galgani, Gaze, Giuliano, Grandjean, Hahn, Hamdoun, Hess, Judson, Laborde, McGlade, Mu, Mustapha, Neira, Noble, Pedrotti, Reddy, Rocklöv, Scharler, Shanmugam, Taghian, Van De Water, Vezzulli, Weihe, Zeka, Raps and Rampal2020). The impacts of polluted seawater create a huge social and economic cost to communities, with pathogens in ocean pollution causing an estimated $19.4 billion (2022 USD) in economic losses annually because of their direct impacts on humans alone (Shuval, Reference Shuval2003).
Microplastics and debris found in wastewater pollution can also form a unique microbial community that is distinct from the surrounding water (Zettler et al., Reference Zettler, Mincer and Amaral-Zettler2013). The microbial community on plastic can include pathogenic microorganisms, such as Vibrio spp., that cause infections through contaminated water or seafood consumption (Zettler et al., Reference Zettler, Mincer and Amaral-Zettler2013; Kirstein et al., Reference Kirstein, Kirmizi, Wichels, Garin-Fernandez, Erler, Löder and Gerdts2016). In the case of some zoonotic parasitic microbes that cause illness in aquatic wildlife and illness in humans from shellfish consumption, counts of the microbes are higher on plastics than in surrounding water (Zhang et al., Reference Zhang, Kim, Rueda, Rochman, VanWormer, Moore and Shapiro2022). Plastics therefore potentially create a novel habitat for pathogens to be concentrated and dispersed beyond their typical range, as floating plastics can travel longer distances than natural substrates (e.g., wood and macroalgae), and sinking microplastics are readily ingested by filter-feeding shellfish (Zettler et al., Reference Zettler, Mincer and Amaral-Zettler2013; Littman et al., Reference Littman, Fiorenza, Wenger, Berry, van de Water, Nguyen, Aung, Parker, Rader, Harvell and Lamb2020; Zhang et al., Reference Zhang, Kim, Rueda, Rochman, VanWormer, Moore and Shapiro2022).
Polluted coastal ecosystems also affect the health of coastal human populations through fisheries decline (Hicks et al., Reference Hicks, Cohen, NAJ, Nash, Allison, D’Lima, Mills, Roscher, Thilsted, Thorne-Lyman and MacNeil2019; Li et al., Reference Li, Tian, Xu and Cheng2019). Millions of people depend on tropical coastal fisheries for essential protein and micro-nutrients (Kawarazuka and Béné, Reference Kawarazuka and Béné2010; Teh et al., Reference Teh, Teh and Sumaila2013). More than 10% of the global population is likely to face micronutrient and fatty acid deficiencies if the current trajectories of fisheries decline continue, especially in the developing nations at the Equator (Golden et al., Reference Golden, Allison, Cheung, Dey, Halpern, McCauley, Smith, Vaitla, Zeller and Myers2016). In addition, individuals already experiencing chronic health effects due to repeated exposure to pathogens will have nutrient absorption challenges, further exacerbating any micronutrient deficiencies from declining fisheries (Chase and Ngure, Reference Chase and Ngure2016). Better recognition of the economic and human health costs resulting from pollution impacts is critical for prioritising action and leveraging the necessary cross-sectoral partnerships and resources required for managing pollution at appropriate scales.
Systems approaches to watershed management
There are an array of site-based management interventions that can be implemented at nested scales within watersheds to improve water quality (Liu et al., Reference Liu, Engel, Flanagan, Gitau, McMillan and Chaubey2017; Richmond et al., Reference Richmond, Golbuu, Shelton, Wolanski, Day, Elliot and Ramachandran2019; Leder et al., Reference Leder, Openshaw, Allotey, Ansariadi, Barker, Burge, Clasen, Chown, Duffy, Faber, Fleming, Forbes, French, Greening, Henry, Higginson, Johnston, Lappan, Lin, Luby, McCarthy, O’Toole, Ramirez-Lovering, Reidpath, Simpson, Sinharoy, Sweeney, Taruc, Tela, Turagabeci, Wardani, Wong and Brown2021). Mitigation efforts typically include policy instruments and place-based interventions.
Policy instruments, such as regulations or market-based incentives, can be applied at any scale and are not necessarily spatially bound within watersheds or aimed at specific watersheds. For example, the implementation of policy instruments can control, reduce and/or prevent pollution through improved use, transport, storage and disposal of chemicals (Taylor et al., Reference Taylor, Pollard, Rocks and Angus2012; Olmstead and Zheng, Reference Olmstead and Zheng2021) and nutrients (UNEP, 2012). Policy instruments can also initiate the implementation of soil conservation and erosion/runoff control strategies, such as maintaining riparian buffer zones by legislating mangrove protection (Richmond et al., Reference Richmond, Golbuu, Shelton, Wolanski, Day, Elliot and Ramachandran2019).
Place-based interventions are specifically applied at a range of scales, from landscape, residential, down to individual and microbial scales (Figure 2). Traditionally, human health focused place-based interventions have been targeted at a residential and individual scale, through the application of water, sanitation and hygiene (WASH) infrastructure improvements or behaviour change campaigns (World Health Organization (WHO), 2016; World Health Organization (WHO) and United Nations Children’s Fund (UNICEF), 2021). However, there is now substantial evidence that landscape scale interventions could deliver significant human health outcomes, while also protecting ecosystem health. For example, a study involving 35 developing countries found that higher upstream tree cover in watersheds was associated with a lower probability of childhood diarrhoeal disease downstream (Herrera et al., Reference Herrera, Ellis, Fisher, Golden, Johnson, Mulligan, Pfaff, Treuer and Ricketts2017). In Hawai ͑i, Ragosta et al. (Reference Ragosta, Evensen, Atwill, Walker, Ticktin, Asquith and Tate2011) demonstrated that higher riparian canopy cover was associated with lower Enterococcus concentrations in stream water. New genomics research is beginning to reveal how more intact ecosystems, from the watershed to the individual organism scale, are more likely to carry lower pathogen loads (Hess et al., Reference Hess, Wenger, Ainsworth and Rummer2015; Shore-Maggio et al., Reference Shore-Maggio, Aeby and Callahan2018; Bass et al., Reference Bass, Stentiford, Wang, Koskella and Tyler2019). Coastal ecosystems also play a key role in regulating disease risk in the marine environment, with a recent study showing that when seagrass meadows are present, there are 50% fewer potentially pathogenic bacteria capable of causing disease in humans and aquatic organisms (Lamb et al., Reference Lamb, Willis, Fiorenza, Couch, Howard, Rader, True, Kelly, Ahmad, Jompa and Harvell2017). However, coastal ecosystems themselves are vulnerable to high levels of pollution (Crain et al., Reference Crain, Halpern, Beck and Kappel2009; Wear, Reference Wear2016; Turschwell et al., Reference Turschwell, Connolly, Dunic, Sievers, Buelow, Pearson, Tulloch, Côté, Unsworth, Collier and Brown2021), underscoring the importance of implementing a system-wide approach when managing watersheds.
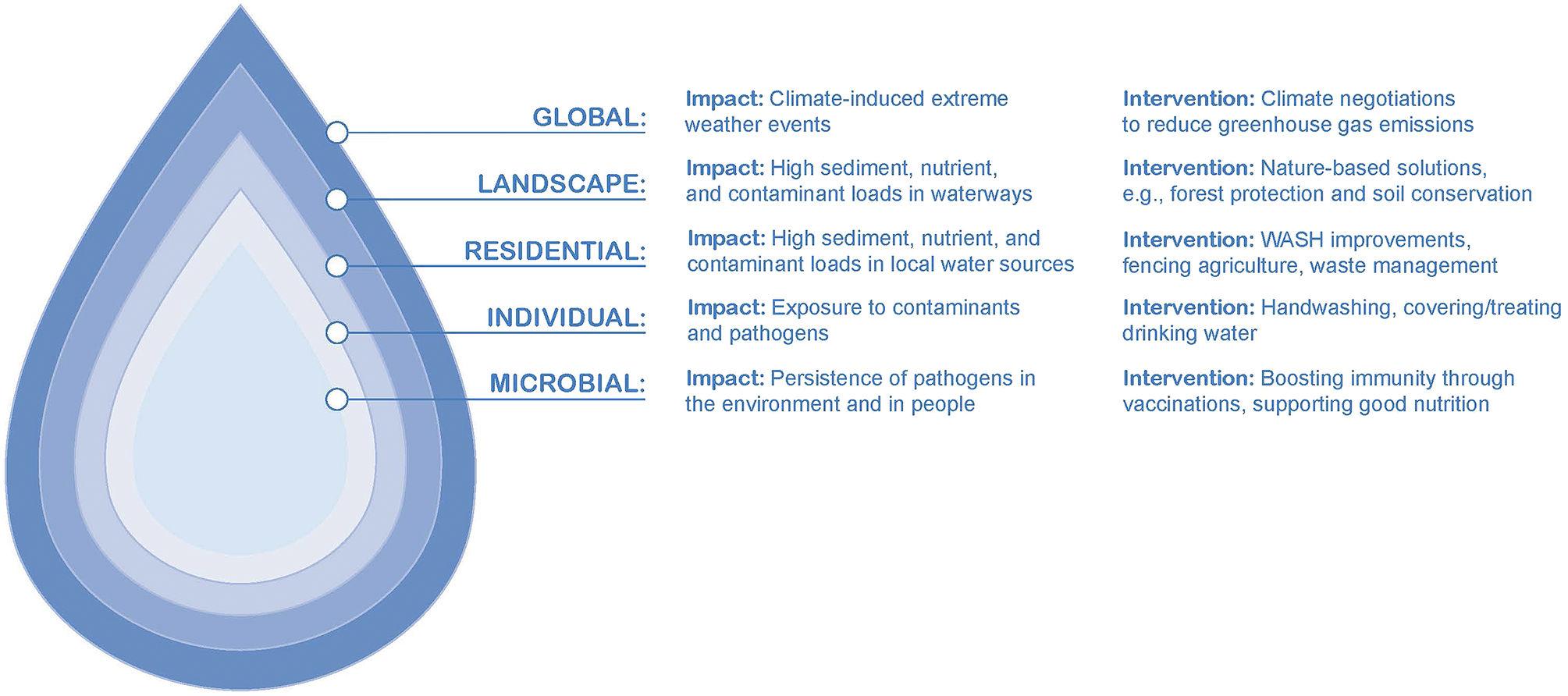
Figure 2. Nested scales of watershed processes.
Despite the recognition that pollution is one of the greatest threats facing coral reef ecosystems (Burke et al., Reference Burke, Reytar, Spalding and Perry2011; Andrello et al., Reference Andrello, Darling, Wenger, Suárez‐Castro, Gelfand and Ahmadia2021), there are limited examples of water quality management associated with successful recovery of coral reef ecosystems, and of those, the management interventions have primarily only tackled pollution arising from point-source pollution (Birkeland et al., Reference Birkeland, Green, Fenner, Squair and Dahl2013; Reef Resilience Network, 2021). Designing and measuring the effectiveness of policy instruments for water quality management is difficult due to lack of compliance and information on contaminant thresholds and monitoring (Taylor et al., Reference Taylor, Pollard, Rocks and Angus2012; Olmstead and Zheng, Reference Olmstead and Zheng2021). Place-based interventions are often impeded by difficulties in engaging stakeholders, lack of systematic/transparent planning, and funding shortfalls (Jupiter et al., Reference Jupiter, Wenger, Klein, Albert, Mangubhai, Nelson, Teneva, Tulloch, White and Watson2017; Ayala-Orozco et al., Reference Ayala-Orozco, Rosell, Merçon, Bueno, Alatorre-Frenk, Langle-Flores and Lobato2018). For example, where stakeholders are not effectively engaged, interventions can be hindered by divergent visions, interests, and tensions within and between sectors (Ayala-Orozco et al., Reference Ayala-Orozco, Rosell, Merçon, Bueno, Alatorre-Frenk, Langle-Flores and Lobato2018). Lack of engagement can also limit buy-in and uptake of interventions by groups (Oteros-Rozas et al., Reference Oteros-Rozas, Martin-Lopez, Daw, Bohensky, Butler, Hill, Martin-Ortega, Quinlan, Ravera, Ruiz-Mallen and Thyresson2015; Mitchell et al., Reference Mitchell, Mitchell, Hunt, Townsend and Lee2022). Lack of systematic/transparent planning and evaluation can generate a lack of trust, accountability and credibility from the perspective of stakeholders (Ayala-Orozco et al., Reference Ayala-Orozco, Rosell, Merçon, Bueno, Alatorre-Frenk, Langle-Flores and Lobato2018), and lead to missed opportunities for effective action (Jupiter et al., Reference Jupiter, Wenger, Klein, Albert, Mangubhai, Nelson, Teneva, Tulloch, White and Watson2017; Beer et al., Reference Beer, McKenzie, Blažek, Sotarauta and Ayres2020). Funding shortfalls and lack of personnel prohibit action at the scale and duration required (Ayala-Orozco et al., Reference Ayala-Orozco, Rosell, Merçon, Bueno, Alatorre-Frenk, Langle-Flores and Lobato2018; Beer et al., Reference Beer, McKenzie, Blažek, Sotarauta and Ayres2020). Interventions for nonpoint source pollution can be particularly challenging as pollution loading is difficult to estimate and is often attributable to many stakeholders and sectors beholden to different regulations (Shortle and Horan, Reference Shortle and Horan2001).
Kāneʻohe Bay in Hawaii is a commonly cited case-study of point-source pollution (sewage) management for coral reef ecosystems resulting in a rarely seen recovery from an algal dominated back to a coral dominated state (Bahr et al., Reference Bahr, Jokiel and Toonen2015). More recent successes include recovery of coral reef ecosystems within Faga’alu Bay in American Samoa and Molokaʻi in Hawai‘i, where harmful runoff from the upstream quarry activities (Samoa) and invasive ungulate species (Hawaiʻi) were managed through targeted watershed interventions (Vargas-Ángel and Huntington, Reference Vargas-Ángel and Huntington2020). Both regions’ intervention strategies required large and costly monitoring efforts to observe success, and both observed setbacks in recovery trajectories due to external disturbances (e.g., storm waves and bleaching; Bahr et al., Reference Bahr, Jokiel and Toonen2015; Vargas-Ángel and Huntington, Reference Vargas-Ángel and Huntington2020).
Watershed case study 1: Watershed interventions for systems health in Fiji
Low coverage of properly treated drinking water and sanitation in remote areas of Fiji leaves communities heavily reliant on the safety and security of unprotected water sources and vulnerable to water-related diseases. Severe outbreaks of water-related infectious diseases, such as leptospirosis, typhoid and dengue (hereafter LTD), are common. LTD cases and associated syndromes are correlated with environmental conditions, with large outbreaks typically occurring following heavy rainfall and flooding (Lau et al., Reference Lau, Smythe, Craig and Weinstein2010; Nelson et al., Reference Nelson, Jenkins, Jupiter, Horwitz, Mangubhai, Abimbola, Ratu, Naivalulevu and Negin2022), with increased severity within degraded watersheds (Jenkins et al., Reference Jenkins, Jupiter, Mueller, Jenney, Vosaki, Rosa, Naucukidi, Mulholland, Strugnell, Kama and Horwitz2016).
Coastal and freshwater ecosystems are also threatened by degraded watersheds in Fiji, with decreased fish, coral and seagrass cover seen downstream of cleared and developed watersheds due to the runoff of harmful pollutants (Jenkins et al., Reference Jenkins, Jupiter, Qauqau and Atherton2010; Brown et al., Reference Brown, Jupiter, Lin, Albert, Klein, Maina, Tulloch, Wenger and Mumby2017; McKenzie and Yoshida, Reference McKenzie and Yoshida2020). These ecosystems support the livelihoods, nutrition and incomes of many rural communities (Mangubhai et al., Reference Mangubhai, Sykes, Lovell, Brodie, Jupiter, Morris, Lee, Loganimoce, Rashni, Lal, Nand, Qauqau and Sheppard2018).
The Watershed Interventions for Systems Health in Fiji (WISH Fiji) project aims to address these overlapping problems through a collaborative effort between government, academic and non-governmental organisations (NGO) partners. Project collaborators are co-designing targeted ‘up-stream’ interventions implemented across various nested scales (Figure 2) with local communities to prevent, detect and respond to LTDs, in addition to mitigating degradation of downstream resources and ecosystems (McFarlane et al., Reference McFarlane, Horwitz, Arabena, Capon, Jenkins, Jupiter, Negin, Parkes and Saketa2019). In doing so, the WISH Fiji project aims to transform both environmental and public health action from reactive to preventative, and improve the overall health of the system to maintain integrity against LTD and natural disasters.
Watershed case study 2: Wastewater management in Roatan, Honduras
Roatan Island, in the Bay Islands of Honduras, is bordered by coral reef ecosystems that attract over a million tourists into the region. Provisioning unpolluted runoff from watersheds is essential to maintaining the health of these ecosystems, but also to protect the health of Roatan communities and tourists. However, limited wastewater treatment on the island resulted in discharge of untreated or inadequately treated wastewater directly onto coral reef ecosystems. Local ecological knowledge linked this wastewater runoff to outbreaks of water-related infectious disease in both humans and corals in the region, which raised fears of impacts on tourism (the main source of income in Roatan).
To combat both the human health and ecosystem impacts of untreated wastewater discharge, a collaboration between government, conservation groups and water associations identified the need for a community wastewater treatment plant (WWTP) and water quality program in West End, Roatan. The West End WWTP was then built in 2011 and has since been connected to 99% of accessible homes and businesses in the area.
Critically, a water quality laboratory led by the Bay Islands Conservation Association was also built to enable testing of marine water downstream of the WWTP, allowing significant improvements in water quality to be observed. Within 7 years of the WWTP installation, the public beach downstream passed the United States EPA safe swimming standards for Enterococcus, a bacteria which can cause a variety of infections and is associated with faecal contamination. The beach has since been awarded an Ecological Blue Flag certification that validates the areas as safe for tourists. Improved metrics for coral reef ecosystem health were also observed, likely as a result of improved water quality (Coral Reef Alliance, 2020).
Key enabling factors
Cross-sectoral coordination and integrated governance
Managing watersheds offers numerous opportunities to address systems health challenges linked to achievement of multiple SDGs (Jenkins et al., Reference Jenkins, Capon, Negin, Marais, Sorrell, Parkes and Horwitz2018a), but simultaneously tackling multiple objectives requires coordination and integrated governance. Cross-sectoral collaborations can create a more holistic understanding of the watershed system and the breadth of its impacts across sectors (Parkes et al., Reference Parkes, Morrison, Bunch, Hallström, Neudoerffer, Venema and Waltner-Toews2010). This holistic understanding can improve the efficiency of integrated watershed management (IWM) by targeting multiple problems at once, creating the potential for win–win scenarios for both coastal ecosystem health and human health (Jupiter et al., Reference Jupiter, Jenkins, Lee Long, Maxwell, Carruthers, Hodge, Govan, Tamelander and Watson2014; Jenkins and Jupiter, Reference Jenkins, Jupiter, Finlayson, Horwitz and Weinstein2015).
The success of cross-sectoral coordination and governance relies on careful participatory engagement and integrated policy development and implementation (Olsen and Christie, Reference Olsen and Christie2000; Lane, Reference Lane2008). Decision-making should be developed through engagement with a wide range of stakeholders and resource users at multiple scales, improving coordination between divisions that may typically focus on the coastline or in specific sectors (Wang et al., Reference Wang, Mang, Cai, Liu, Zhang, Wang and Innes2016). Care should be taken to incorporate information from multiple knowledge systems in planning and practice to ensure alignment with local values and objectives (Tengö et al., Reference Tengö, Brondizio, Elmqvist, Malmer and Spierenburg2014). Engagement should capture the diversity of land and water use practices, needs, goals and potential conflicts across sectors, and ensure that all involvement is participatory, transparent, accountable and culturally appropriate (Jupiter et al., Reference Jupiter, Jenkins, Lee Long, Maxwell, Carruthers, Hodge, Govan, Tamelander and Watson2014; Richmond et al., Reference Richmond, Golbuu, Shelton, Wolanski, Day, Elliot and Ramachandran2019).
Managing watersheds for systems health often requires coordination across multiple jurisdictions and administrative units that operate within and beyond watershed boundaries. Watershed governance is thus complicated by the mismatched boundaries of biophysical processes operating within drainage basins and jurisdictional boundaries of administrative systems responsible for land use policy implementation and health systems surveillance and delivery (Davidson and De Loë, Reference Davidson and De Loë2014). Polycentric and collaborative governance approaches, particularly those involving Indigenous peoples and local communities, are appropriate in this context to bridge across sectors and jurisdictional levels and address watershed systems issues at appropriate scales (e.g., Huitema et al., Reference Huitema, Mostert, Egas, Moellenkamp, Pahl-Wostl and Yalcin2009; Morrison, Reference Morrison2017). Watershed management across multiple agencies and organisations can be coordinated by specific institutions that can serve as bridging organisations, such as catchment authorities, which operate most effectively when they have legislated mandates and operating budgets (Parkes et al., Reference Parkes, Morrison, Bunch, Hallström, Neudoerffer, Venema and Waltner-Toews2010; Davidson and De Loë, Reference Davidson and De Loë2014).
Critically, integrated policy needs to be developed based on a good understanding of the connections among systems so that evidence-based predictions and decisions can be made about how any interventions may influence outcomes in multiple sectors (Álvarez-Romero et al., Reference Álvarez-Romero, Adams, Pressey, Douglas, Dale, Augé, Ball, Childs, Digby, Dobbs, Gobius, Hinchley, Lancaster, Maughan and Perdrisat2015). It is essential to consider any potential trade-off scenarios wherein mutual benefits are not shared between sectors, or one sector may even be exposed to more harm. For example, the construction or restoration of wetlands for improving water quality and ecosystem health may have unintended consequences for mosquito-borne disease risk (Malan et al., Reference Malan, Appleton, Day and Dini2009; Horwitz and Finlayson, Reference Horwitz and Finlayson2011); and the installation of dams and weirs for improving water security and sediment pollution may have unintended consequences for freshwater ecosystems and fisheries (Dudgeon et al., Reference Dudgeon, Arthington, Gessner, Kawabata, Knowler, Lévêque, Naiman, Prieur-Richard, Soto, Stiassny and Sullivan2006; Kroon et al., Reference Kroon, Schaffelke and Bartley2014). Having a wide range of informed stakeholders sharing resources and taking an integrated approach will assist in buffering this risk and create more effective and proactive governance wherein benefits across sectors are optimised.
Sustainable financing
Improving water quality through upstream interventions is expensive and requires sustained investment (Muchapondwa et al., Reference Muchapondwa, Stage, Mungatana and Kumar2018). There is often a long lag time between implementing interventions and observing improvements in metrics of ecosystem and public health, while success can also be obscured by other disturbances, such as cyclones and coral bleaching (Richmond et al., Reference Richmond, Golbuu, Shelton, Wolanski, Day, Elliot and Ramachandran2019). Delays in realising anticipated benefits create disincentives for long-term action when program and policy targets require short-term results.
Water and watershed funds are a common financing tool used in various geographies globally to ensure a sustained source of funding (The Nature Conservancy (TNC) and Goldman, Reference Goldman2009; Kauffman, Reference Kauffman2014). These funds are often resourced through voluntary contributions of donors and water users, such as utility companies and farmers, which are then used to pay for and support upstream strategies to conserve the quality and security of water sources. Boards may invest the funding directly or use grants to identify and develop critical intervention strategies (The Nature Conservancy (TNC) and Goldman, Reference Goldman2009). Linking the needs of downstream water users with upstream communities and land users allows the funds to provide a low-cost and sustainable financing method of maintaining clean and regular water supply (The Nature Conservancy (TNC) and Goldman, Reference Goldman2009).
Examples of successful water funds are mainly from temperate regions and exclude marine ecosystems, such as the Latin American Water Funds Partnership (LAWFP). LAWFP is an agreement between a consortium of international NGOs to enhance and preserve water security in Latin America and supports 25 water funds across nine countries with varying water management goals and local funding bodies (Bremer et al., Reference Bremer, Auerbach, Goldstein, Vogl, Shemie, Kroeger, Nelson, Benítez, Calvache, Guimarães, Herron, Higgins, Klemz, León, Sebastián Lozano, Moreno, Nuñez, Veiga and Tiepolo2016). In total, LAWFP supported water funds are managing over 227,000 ha of land, potentially benefiting 89 million people, and have leveraged over $205 million USD in resources. Many funds prioritise not only water infrastructure management for humans, but also the use of nature-based solutions as a means to preserve the health of aquatic ecosystems (Kauffman, Reference Kauffman2014). However, as with many water funds (and conservation efforts), there have been limited measurements of the outcomes or baselines to fully perceive the benefits of these funds (Bremer et al., Reference Bremer, Auerbach, Goldstein, Vogl, Shemie, Kroeger, Nelson, Benítez, Calvache, Guimarães, Herron, Higgins, Klemz, León, Sebastián Lozano, Moreno, Nuñez, Veiga and Tiepolo2016).
The availability of local sources of funding for sustainable financing of a water or watershed fund will vary from region to region as beneficiaries vary. Not all communities and industries pay for water use: under these circumstances, it may be feasible to develop business cases for investment based on foregone healthcare and productivity costs if watershed improvements prevent people from getting sick. Key to developing these business plans is first assessing how much disease risk can be reduced by a portfolio of management interventions and balancing the wide range of savings in foregone costs (healthcare, missed work and education, tourism impacts) against annual investment needs. Considerations also need to be taken for the potential benefits from buffering against the influence of climate change on disease.
Various other types of conservation and climate change financing can additionally or alternatively support watershed management financing. For example, in some coral reef areas, payment for ecosystem services schemes have also been proposed as a way for downstream resource users to incentivise upstream resources users to manage water quality (Goldman-Benner et al., Reference Goldman-Benner, Benitez, Boucher, Calvache, Daily, Kareiva, Kroeger and Ramos2012; Peng and Oleson, Reference Peng and Oleson2017). Climate financing that supports nature-based solutions is commonly expected to deliver various water services, though evidence shows mixed results on base flow, annual surface runoff and water quality depending on local geographic conditions and the mix of interventions utilised (Vigerstol et al., Reference Vigerstol, Abell, Brauman, Buytaert, Vogl, Cassin, Matthews and Gunn2021; de Freitas et al., Reference de Freitas, de Moraes, da Costa, Martins, Silva, Avanzi and Uezu2022).
Conclusions/recommendations
The latest science makes it clear that unplanned development, poor land use, unsustainable agricultural practices and poor wastewater management within watersheds are significant threats to coastal populations and ecosystems. Despite the threats, incentivising improved watershed management practices for the sake of improving water quality for downstream environmental benefits has remained a challenge. In the future, it is recommended that policies and management are designed using systems health approaches that aim to restore water quality to achieve multiple benefits for human and coastal ecosystem health, while facilitating sustainable social and economic development.
Through our review, we identified a series of actionable recommendations to promote holistic approaches to watershed management for systems health (Table 3). These include best practice lessons from existing, IWM programs on: inclusive planning; implementation through cross-sectoral coordination; participatory management; monitoring to identify risks and measure progress of interventions; mobilising resources to sustain long-term action; and sharing information to promote replication and scaling of integrated approaches. To achieve SDG targets by 2030, there is increasing urgency to prioritise these types of management approaches that simultaneously deliver on benefits for nature, people and climate.
Table 3. Recommendations for planning, coordinating, monitoring, resourcing and scaling sustained investment in integrated watershed management for systems health

Open peer review
To view the open peer review materials for this article, please visit http://doi.org/10.1017/cft.2023.15.
Data availability statement
No data presented in this review.
Acknowledgements
Sarah Markes provided graphic design for Figure 1 and Haley Williams provided graphic design for Figure 2.
Author contribution
S.D.J. created the ideas and outline for the manuscript. All authors reviewed outline and provided feedback on the format and scientific content of the outline. A.Wa., S.J., A.We. and A.J. created the first written draft of the manuscript. A.Wa. edited the figures and created the tables. J.L., C.D.K., D.C., C.C., K.F., A.R. and H.S.G. provided critical revision of the scientific content of the manuscript and provided stylistic/grammatical revisions, over multiple drafts. A.Wa. addressed revisions and compiled the final manuscript.
Financial support
Manuscript development was supported by grant #53006 from Bloomberg Philanthropies to the Wildlife Conservation Society under the Vibrant Oceans Initiative.
Competing interest
No competing interest to declare.
Comments
Prof. Tom Spencer
Editor-in-Chief
Cambridge Prisms: Coastal Futures
Dear Dr Spencer,
We wish to submit a standard review article entitled “Integrated watershed management solutions for healthy coastal ecosystems and people” to be considered for publication in your Cambridge Prisms: Coastal Futures journal. This review article was originally commissioned on the 6th of April 2022 by your senior editor, Martin Le Tissier, to be published with the journal’s launch in January 2023.
In this review, we provide a novel summary of the overlapping impacts of water pollution on both human and coastal ecosystem health, focusing primarily on the tropics and coral reef ecosystems. We use this information to identify holistic approaches to integrated watershed management that target overlapping drivers of ill-health in downstream coastal ecosystems and people. We provide recommendations of key actions for integrated watershed management that can help achieve multiple Sustainable Development Goals for both nature and people on coasts.
Given the extensive and multidisciplinary impacts of water pollution on coastal environments globally, we believe the summary and recommendations provided in our article will appeal to the future readership of Cambridge Prisms: Coastal Futures. Despite the extensive impacts of poor water quality, previous pollution control methods have been highly sectorised and under-resourced, with poor coordination of implementation, often across insufficient scales to realise benefits. This review will allow your readers to understand the cross-sectoral impacts and drivers of water pollution, and provide evidence-based management actions to support planning and decision-making for a wide range of stakeholders in coastal environments from government, civil society, and the private sector.
Please address all correspondence concerning this manuscript to me at <u>[email protected]</u>.
Thank you for your consideration of this article.
Sincerely,
Ama Wakwella, PhD Candidate
School of Earth and Environmental Sciences
Centre for Biodiversity and Conservation Science
The University of Queensland
<u>[email protected]</u>
Co-author
Dr Amelia Wenger
Wildlife Conservation Society, Global Marine Program, Bronx, NY 10460, USA
<u>[email protected]</u>
Co-author
Dr Aaron Jenkins
The University of Sydney, Sydney School of Public Health, Sydney Institute for Infectious Diseases, Camperdown NSW 2006, Australia
Edith Cowan University, School of Science, Centre for People Place and Planet, Joondalup WA 6027, Australia
<u>[email protected]</u>
Co-author
Asst. Prof Joleah Lamb
University of California - Irvine, Department of Ecology and Evolutionary Biology, Irvine, CA 92657, USA
<u>[email protected]</u>
Co-author
Dr Caitlin Kuempel
Australian Research Council Centre of Excellence for Coral Reef Studies, University of Queensland, St Lucia QLD 2072, Australia
Griffith University, School of Environment and Science, 170 Kessels Road, Nathan QLD 4111, Australia
<u>[email protected]</u>
Co-author
Dr Danielle Claar
Washington State Department of Natural Resources, Olympia, WA, USA
<u>[email protected]</u>
Co-author
Dr Chris Corbin
United Nations Environment Programme, Cartagena Convention Secretariat, Kingston, Jamaica
<u>[email protected]</u>
Co-author
Dr Kim Falinski
The Nature Conservancy, Hawai`i and Palmyra Chapter, Honolulu, Hawaii, USA
<u>[email protected]</u>
Co-author
Dr Antonella Rivera
The Coral Reef Alliance, Mesoamerican Region, 548 Market Street, Suite 29802, San Francisco, CA 94104-5401, USA
<u>[email protected]</u>
Co-author
Assoc. Prof Hedley Grantham
Bush Heritage Australia, Melbourne, VIC 3000, Australia
University of New South Wales, Centre for Ecosystem Science, Sydney, NSW 2052, Australia
<u>[email protected]</u>
Co-author
Dr Stacy Jupiter
Wildlife Conservation Society, Melanesia Program, Suva, Fiji
<u>[email protected] </u>